1-10 microns PLGA microsphere production using the RayDrop
Polymeric microparticles, especially PLGA microspheres, are gaining prominence in the drug delivery field due to their biodegradability. Microfluidics offers precise control for PLGA microsphere synthesis. With its unique design, the RayDrop (designed and developed by Secoya Technologies) overcomes the challenges of classic chips. Previous work showcased its ability to synthesize PLGA microspheres (15-50 µm), and now, as demonstrated in this application note, its capacity to produce PLGA microspheres in the 1 to 10 µm range, highlighting versatility in size control.
The RayDrop, developed and manufactured by Secoya Technologies, is used in this application note.
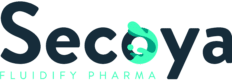
What are PLGA Microspheres?
In the pharmaceutical field, progress for effective disease treatment requires the precise delivery of drugs, vaccines, genes, and various biomolecules to specific sites while simultaneously ensuring the stability and safety of these agents. Ongoing research has long explored new drug delivery formulations, with a current emphasis placed on polymeric microparticle systems and their advantages.
Poly (lactic-co-glycolic acid) or PLGA microspheres have gained interest as controlled drug release carriers in the pharmaceutical and biomedical field due to their favorable properties such as biodegradability, biocompatibility, controllable drug release profiles, and surface modification capabilities for targeted treatment. As a result, PLGA microbeads can be already found in various applications and research works, including cancer, cardiovascular diseases, neurological disorders, dentistry, orthopedics, vaccine therapy, theranostics, and emerging diseases like COVID-19 (Figure 1).1,2
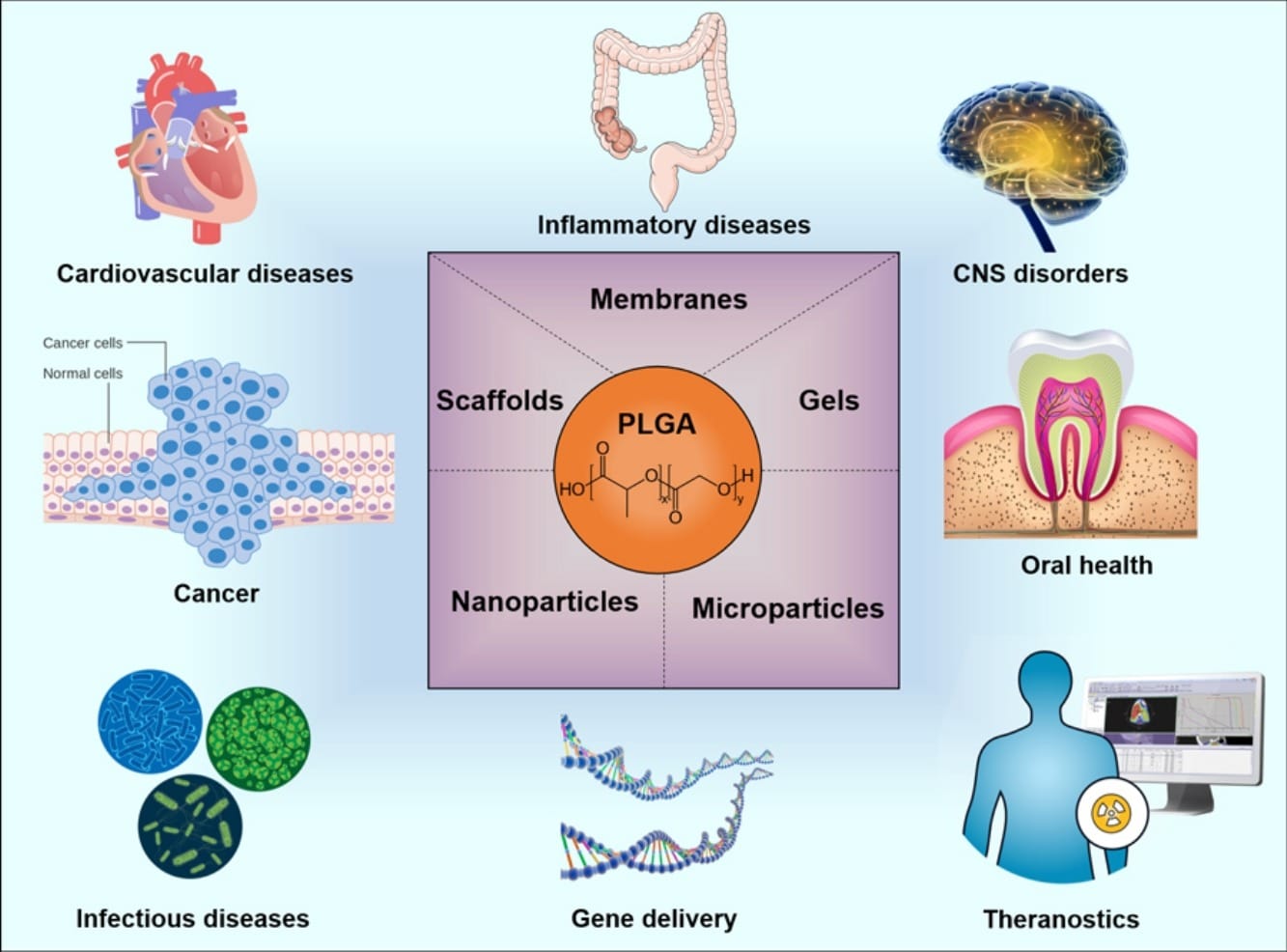
Figure 1. Example of some applications where PLGA can be used for drug delivery. [1]
What are the traditional methods of PLGA formation?
Various techniques can be applied for the preparation of microparticles in drug delivery applications, including physicochemical, chemical, and mechanical processes leading to a great variety of morphologies, structures, and size ranges (Figure 2).3
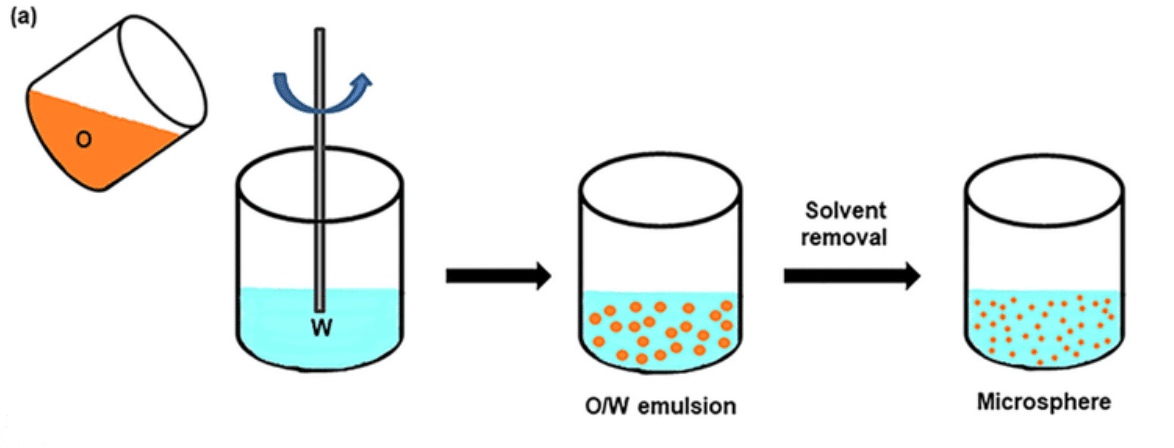
Figure 3. Schematic of PLGA microspheres prepared via emulsification. [4]
Even though emulsification–solvent evaporation is a simple, low-cost, and fast technique, it presents a low encapsulation efficiency and a polydispersity of the PLGA microsphere size. In addition, the encapsulation of hydrophilic agents often requires double or multiple emulsions, which pose drawbacks using the emulsification-solvent evaporation process such as the need for large amounts of organic solvents and challenges in scaling up (Figure 3).4
Other strategies like spray drying and electrospray offer appealing approaches for microencapsulation (Figure 4). The process involves spraying a solid-in-oil dispersion or water-in-oil emulsion in a stream of heated air to produce drug-loaded microspheres.
While this method is effective in encapsulating various drugs/proteins without significant loss of biological activity, a major drawback is the adhesion of microparticles to the inner walls of the spray dryer.
Additionally, controlling particle size can be challenging, and yields for small batches are moderate.4,5
Microfluidic technologies emerge as a powerful tool for PLGA microsphere synthesis, enabling a precise generation of microbeads with high monodispersed, tunable structures, and excellent encapsulation efficiency. Unlike bulk emulsification methods, microfluidic devices generate emulsions by fabricating one drop at a time between two immiscible liquid phases. This process results in highly monodisperse emulsion droplets, offering precise control over the size of the final polymer microparticles. Despite the limited production scale in a single microfluidic device, scaling up is achievable by simultaneously operating multiple devices in parallel.3,4,5
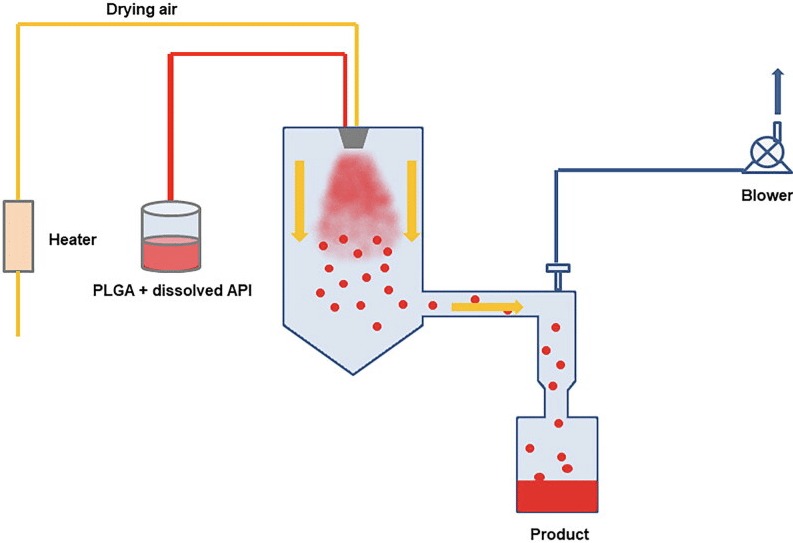
Figure 4. Diagram of the spray drying process. [4]
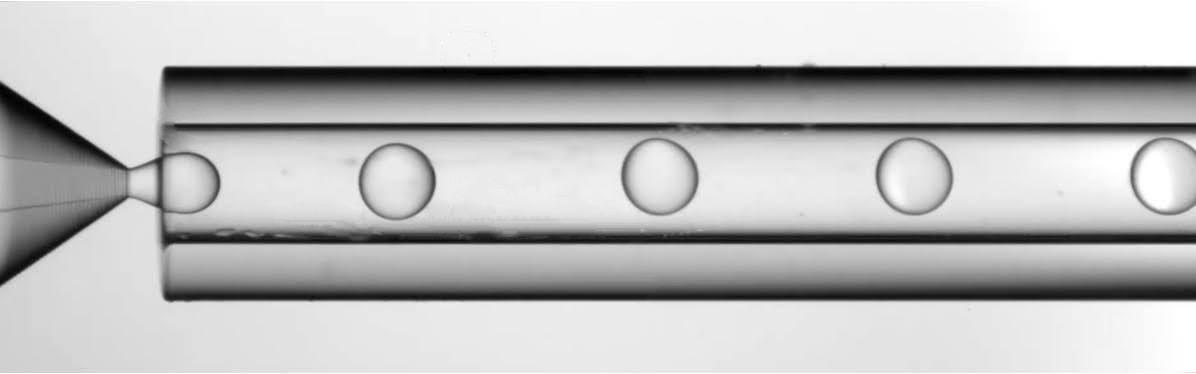
Figure 5. Ethyl Acetate – PLGA droplet production with the RayDrop.
Yet a prolonged multi-hour operation for PLGA microparticle production remains challenging to achieve with classical chips due to their planar geometry, especially for low-size PLGA microspheres (1-10 µm). Secoya Technology’s RayDrop addresses planar microfluidic limitations by employing two cylindrical capillaries in a metallic chamber6. One capillary injects droplets through a 3D-printed nozzle, while the other collects droplets. The cylindrical geometry prevents contact with capillary walls, avoiding wetting issues and ensuring high-quality microfluidics.
Method | Advantages | Drawbacks |
---|---|---|
Emulsification solvent – evaporation | – Easy scaling-up – Certain ability to control particle size | – Biomacromolecule instability – Batch-to-Batch variance – Polydispersity of particle size |
Spray-drying | – Fast and convenient – Suitable for OEM scaling up – Less harsh conditions for proteins | – Adhesion of the microspheres to the inner walls of the spray dryer – Difficulty in control of size |
Microfluidics | – Precise of processing parameters – Ease of fabricating double, triple, and even higher-order emulsions | – Instrument dependent – Relatively low yield |
Table 1. Advantages and drawbacks of microparticule production techniques. [5]
How to produce PLGA microspheres (1-10 microns) using a single emulsion device, the RayDrop
Our previous application note regarding the synthesis of PLGA microparticles already demonstrated the capabilities of the RayDrop to produce PLGA microspheres with diameters between 15 and 50 µm. In comparison to other technologies available on the market, the PLGA microbead production pack enables great reproducibility and significantly increased monodispersity (CV 2%) (Figure 6). It enables uninterrupted, long-term production of PLGA microparticles for use in investigations.7,8
However, the PLGA microspheres produced had a minimal diameter of 15 µm. This minimal microparticle diameter is due to the only RayDrop geometry available at the time of redaction: The 30-150 µm (inlet nozzle diameter – collection capillary diameter). As new RayDrop geometries can generate smaller droplets than the 30-150 version, it is expected that the RayDrop can now produce smaller PLGA microbeads.
Therefore, this application note demonstrates the capability of the RayDrop single emulsion device to produce PLGA microspheres in the range of 1 to 10 µm and that any formulation developed with one specific RayDrop configuration can directly be used with another configuration to achieve different particle sizes.
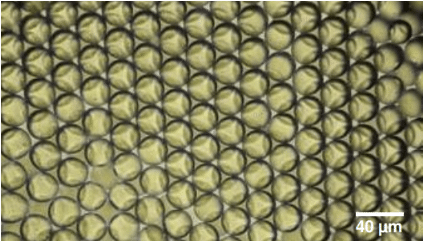
Figure 6. Microscope observation of PLGA microparticles produced by the RayDrop 30-150 µm
PLGA Fabrication Materials
Reagents
The reagents used for the PLGA microsphere production are: deionized water, PLGA Resomer RG 755 s, Poly(vinyl alcohol) (MW13000-23000, 87-89%, hydrolyzed) and Ethyl Acetate (purity 99.7%). All reagents were purchased from Sigma-Aldrich.
The composition of the different phases used are:
Continuous phase | Droplet phase priming & cleaning | Droplet phase production |
---|---|---|
Water+1% PVA | Ethyl Acetate | Ethyl Acetate+2% PLGA |
Microfluidic Setup
A RayDrop with a 30 µm inlet nozzle and a 45 µm counter nozzle is used to perform the PLGA microsphere generation (Figure 7). Its functionality is based on aligning two capillaries within a pressurized chamber containing the outer phase. The inner phase exits through a 3D-printed nozzle positioned in front of a second capillary, where it becomes enveloped by the outer phase. This coflow-focusing approach deviates from the conventional embedded approach and facilitates the creation of a hydrodynamically focused 3D stream. This unique design eliminates wettability issues observed in classical microfluidic chips.
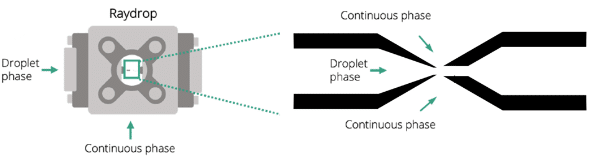
Figure 7. Simplified structure of the Raydrop
The RayDrop (30-45) was installed in the Complex Emulsion Production Platform for easy fluids and droplet flow, control, and imaging. The platform is divided into three parts: mechanical, fluidic, and optical (Figure 8).
The mechanical assembly includes different displacement plates. These allow one to adjust the camera by moving it in x, y, and z directions. It is also possible to position the RayDrop to optimize the visibility of the nozzles on the screen.
The fluidic part contains all the pressure-driven controllers, tubing, and valves necessary for the circulation of fluids. Fluigent’s FlowEZ and Flow Units enable real-time control and measurement of the flow rates, and transitioning from pressure control to flow rate control becomes feasible. Our pressure-driven controllers permit high flow stability and a fast flow change response. This capability enables the continuous production of highly uniform droplets over an extended duration. Also, it is possible to easily set a different pressure or flow rate for each phase.
The optical section contains an LED light source and a color USB 3.0 camera. This camera is connected to a computer to observe droplet formation in real time, as well as to control the stability of the emulsion and measure the size of the generated droplets.
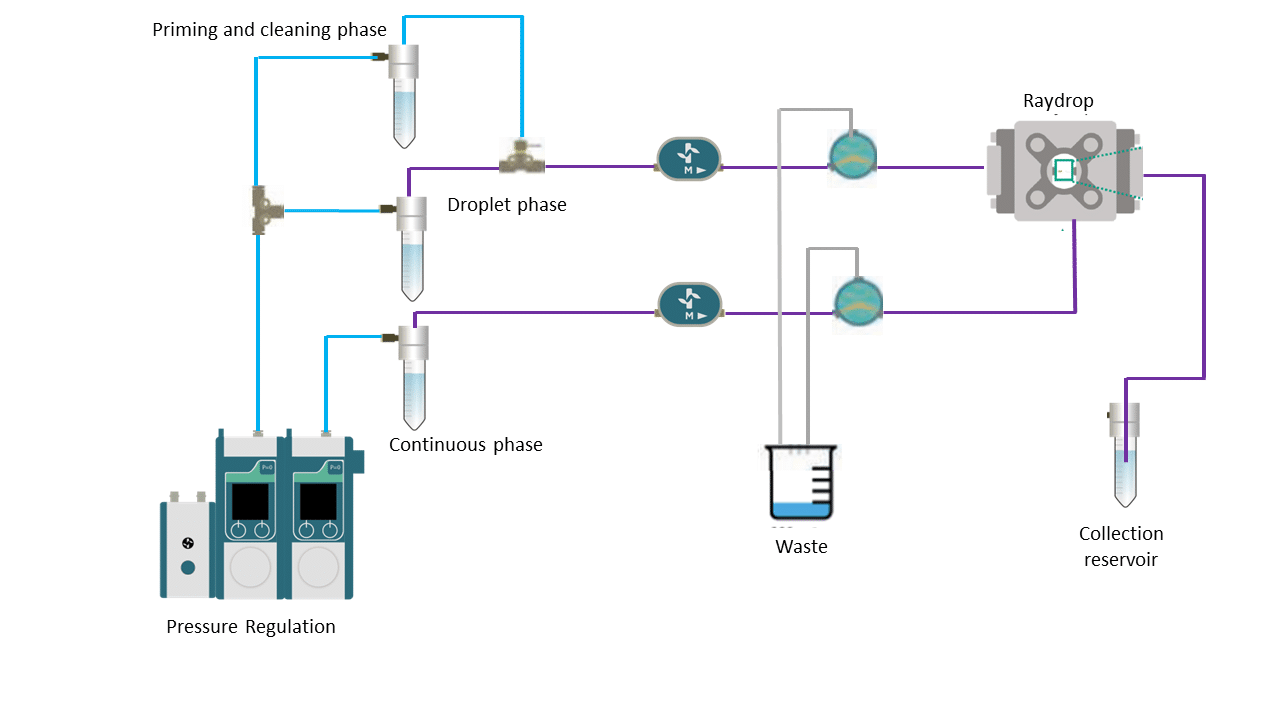
Figure 8. Simplified microfluidic circuit of the Complex Emulsion Production Platform for PLGA microparticle synthesis.
The preparation process of PLGA microspheres
To generate droplets easily, the system must first be started with pure solvent in the droplet phase (Ethyl Acetate). Once the droplet formation is stabilized, the droplet phase is switched to the solution containing the PLGA. This avoids possible clogging issues during the transient phase.
Once the PLGA solution traverses the tubing and reaches the RayDrop, a single emulsion with PLGA droplets is produced in the aqueous continuous phase (Figure 9). The emulsion’s properties are created by adjusting flow rates through Fluigent’s software, Oxygen, to achieve the desired droplet diameter.
The PLGA-Ethyl Acetate droplets can be collected by immersing the tip of the outlet tubing in a small bath of deionized water.
Before concluding the experiment, the cleaning of the RayDrop is done by flushing the droplet phase tubing and the RayDrop nozzle with Ethyl Acetate to dissolve and remove the PLGA, ensuring tubing cleanliness and avoiding clogging issues.
Finally, the PLGA-Ethyl Acetate droplets were left for 5 minutes to complete the polymerization process and were observed with the EvosXL microscope and a 40x magnification.
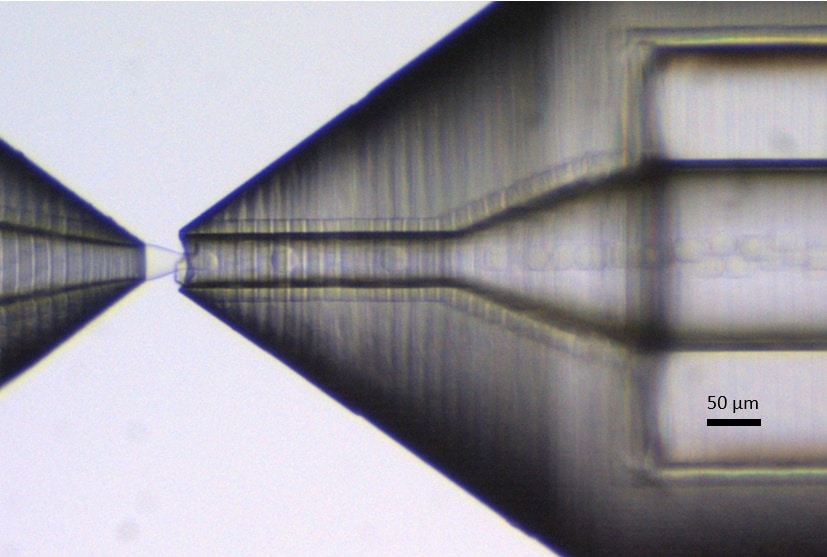
Figure 9. PLGA-Ethyl Acetate droplets in the counter nozzle.
Results: Small microsphere formation with a high monodispersity
Table 2 summarizes the flow rates used to generate the PLGA-Ethyl Acetate droplets and the size of the droplets generated. The RayDrop produces highly monodisperse droplets (21.3 µm mean diameter) with a CV<2% (Figure 10).
Pressure (mbar) | Flowrate (µL/min) | |
---|---|---|
Continuous phase (Water+1% PVA) | 400 | 35 |
Droplet phase (EthylAcetate+2% PLGA) | 80 | 1.8 |
Mean diameter (µm) | CV (%) | |
EthylAcetate+2% PLGA Droplet size | 21.3 | 1.5 |
PLGA microsphere size | 6.9 | 2.4 |
Table 2. PLGA-Ethyl Acetate droplet production and PLGA microsphere production.
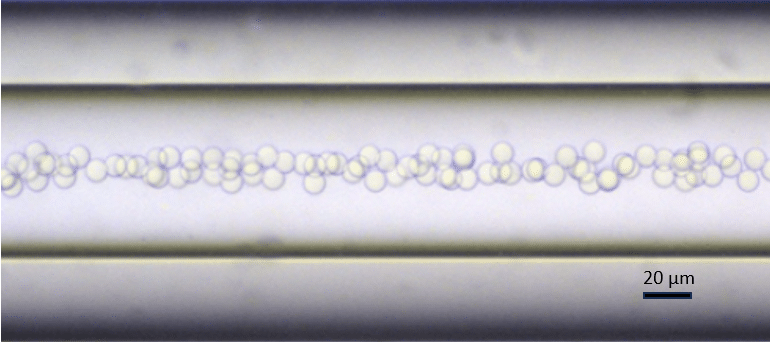
Figure 10. PLGA-Ethyl Acetate droplets in the counter nozzle.
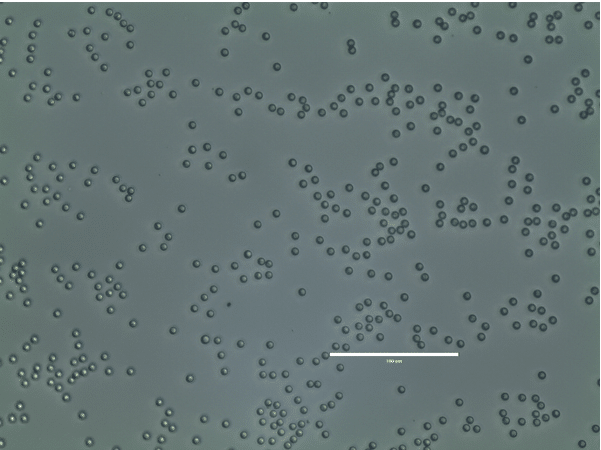
Figure 11. PLGA microparticles observed with an EvosXL and 40x magnification objective. The size bar measures 100 µm.
After collection, ethyl acetate starts to gradually diffuse into the surrounding aqueous solution. Thus, PLGA undergoes precipitation, transforming the droplet into a solid bead. The solvent removal process leads to a reduction in droplet volume, resulting in the formation of a solid bead with a final fixed size.
The figure below presents the produced PLGA microparticles.The PLGA microspheres present a mean diameter of 6.9 µm with a high monodispersity (CV=2.4%) (Figure 11).
Conclusion
The present proof of concept demonstrates the capabilities of the RayDrop to produce PLGA microspheres in the range 1-10 µm with a mean diameter of 6.9 µm. As expected, the combination of a known formulation1,2 with the correct RayDrop allows the production of the desired microsphere size. These results extend the size range of PLGA particles that can be produced efficiently with the RayDrop from nanoparticles, microspheres, and microcapsules.
Related Products
Expertises & Resources
-
Microfluidics Article Reviews Solid lipid nanoparticles for biologics and drug encapsulation Read more
-
Microfluidics White Papers Double emulsion for the generation of microcapsules – a Review Read more
-
Microfluidic Application Notes PLGA nanoparticle synthesis using 3D microfluidic hydrodynamic focusing Read more
-
Microfluidic Application Notes PLGA microcapsules synthesis Read more
-
Microfluidics White Papers Droplet-based Microfluidics – A Complete Guide Read more
-
Expert Reviews: Basics of Microfluidics Microfluidics for vaccine development Read more
-
Microfluidic Application Notes Liposome Nanoparticle Synthesis Read more
-
Microfluidic Application Notes PLGA Microparticles Synthesis Read more
References
- Y.R. Chavan, S.M. Tambe, D.D. Jain, S.V. Khairnar, P.D. Amin, Redefining the importance of polylactide-co-glycolide acid (PLGA) in drug delivery, Annales Pharmaceutiques Françaises, Volume 80, Issue 5, 2022, Pages 603-616;
- Chong Li, Jiancheng Wang, Yiguang Wang, Huile Gao, Gang Wei, Yongzhuo Huang, Haijun Yu, Yong Gan, Yongjun Wang, Lin Mei, Huabing Chen, Haiyan Hu, Zhiping Zhang, Yiguang Jin, Recent progress in drug delivery, Acta Pharmaceutica Sinica B, Volume 9, Issue 6, 2019, Pages 1145-1162, 2211-3835.
- Vlachopoulos, A.; Karlioti, G.; Balla, E.; Daniilidis, V.; Kalamas, T.; Stefanidou, M.; Bikiaris, N. D.; Christodoulou, E.; Koumentakou, I.; Karavas, E.; Bikiaris, D. N. Poly(Lactic Acid)-Based Microparticles for Drug Delivery Applications: An Overview of Recent Advances. Pharmaceutics 2022, 14 (2), 359.
- Su, Y.; Zhang, B.; Sun, R.; Liu, W.; Zhu, Q.; Zhang, X.; Wang, R.; Chen, C. PLGA-Based Biodegradable Microspheres in Drug Delivery: Recent Advances in Research and Application. Drug Delivery 2021, 28 (1), 1397–1418.
- Dawei Ding, Qingdi Zhu, Recent advances of PLGA micro/nanoparticles for the delivery of biomacromolecular therapeutics, Materials Science and Engineering: C, Volume 92, 2018, Pages 1041-1060, 0928-4931.
- Dewandre, A.; Rivero-Rodriguez, J.; Vitry, Y.; Sobac, B.; Scheid, B. Microfluidic Droplet Generation Based on Non-Embedded Co-Flow-Focusing Using 3D Printed Nozzle. Sci Rep 2020, 10 (1), 21616.
- Application note: PLGA MICROPARTICLE SYNTHESIS, Fluigent
- Application note: PLGA MICROCAPSULE SYNTHESIS, Fluigent