UV-Crosslinking of Microparticles
Polymer microparticles find numerous applications in diagnostics, drug analysis, and delivery, or DNA purification. For such applications, precise control over the microparticle size distribution is often required for improving repeatability. Size, for instance, impacts the speed at which drugs or chemicals are delivered and released as well as the dosage.
This application note demonstrates how, by combining Fluigent's pressure-based flow controllers with the RayDrop Double Emulsion developed and manufactured by Secoya, it is possible to obtain a highly reproducible and stable UV-crosslinking of microcapsules process.
Secoya developed and manufactured the RayDrop used to perform this application note.
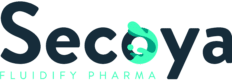
Comparison with other methods
Why use microparticles ?
Microparticles with sizes ranging from 1 μm to 1000 μm have emerged as advanced functional materials for a wide range of biomedical applications, such as drug delivery, tissue engineering, biosensing, and cellular life science. These applications of microparticles depend on their properties which correlate with their size, structure, composition and configuration. Therefore, it is essential to fabricate microparticles in a controlled manner to improve their pharmaceutical capability and reliability for biological studies.
Limitations of standard methods
Conventional methods for microparticle production include solvent evaporation, emulsion polymerization, dispersion polymerization, and spray drying1. These usually result in microparticles with large polydispersity, poor reproducibility, limited functionality, and less tunable morphology2.
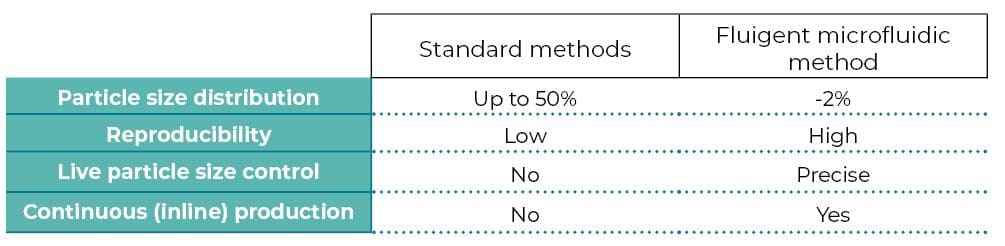
Benefits of droplet microfluidics for microparticles production
To overcome these limitations, droplet microfluidic technology has been implemented. This offers greater control over multiple fluid flows at the microscale. It permits the generation of particles or emulsions with higher monodispersity (~ 5% CV) and complex shapes and structures. Many emulsion droplets can be polymerized (thus usually solidified) upon UV irradiation. This last step can be achieved in a microfluidic system, typically through the downstream channel, where droplets are irradiated with UV light (UV-Crosslinking of Microparticles).
Perform an ultraviolet crosslinking of microparticles
Microfluidic System for micro-sized particles production
Software
Reagents
Continuous phase and liquid for spacing:
- Distilled water with 1% Tween 20
DISPERSED PHASE
- Radcure Generic 7 – A non commercially available resin. However, any type of biocompatible UV polymerized polymer can be used using the same method
- Ethyl acetate 50%
- Photoinitiator TPO 1% (peak absorbance at 395 nm)
Experimental procedure for UV-crosslinking of microparticles
Monodisperse polymer particle synthesis is performed in 3 main steps:
- Generation of monodisperse droplet
- Droplet spacing
- Droplet solidification by UV irradiation
The UV-crosslinking of microparticles processis splitted in three steps: droplet generation, droplet spacing and microcapsule polymerization.
Droplet generation
For droplet generation, two pressure-based controllers (Flow EZ, 2 bar) are connected to two P-CAP reservoirs containing the polymeric phase (dispersed phase) and distilled water with 1% Tween 20 (continuous phase). The reservoirs are connected to the inlets of the Raydrop using tubing, which passes through Flow Units M for flow rate measurement and control. The dispersed phase is injected through the inner capillary of the Raydrop with a flow rate of 5 µL/min, and the continuous phase is delivered to the Raydrop chamber at a flow rate of 25 µL/min. At the intersection between the two capillaries within the Raydrop, droplets are generated (figure 3). Using the above flow rates, droplets are produced with a frequency of 1 Hz and with an outer diameter of about 100 µm.
Droplet spacing
Once generated, droplets exit the Raydrop device with decreased flow velocity (due to the difference between the inner diameter of the downstream channel of the Raydrop and the exit tubing). To avoid clogging during the ultraviolet crosslinking of microparticles, increasing the spacing between droplets is highly recommended. Spacing between droplets can be performed by injecting liquid into the outer tubing in a co-flow manner.
To do so, a spacing device (a T-junction) is added to the outlet tubing (figure 1). Distilled water with 1% Tween 20 (same as the continuous phase) is injected into the spacing device with a flow rate of 250 µL/min.
Droplet polymerization: particle solidification
After being spaced, the droplets are exposed to UV light with an irradiance of 95-100 mW /cm², allowing droplet polymerization and subsequent particle solidification.
Results: Observation of the beads produced
Figure 4: Polymerized beads observed under SEM
After UV-crosslinking of microparticles, beads are recovered in a petri dish and analyzed under a microscope. Figure 4 shows the polymerized beads after synthesis. We can observe beads with an average diameter of 83 µm, and narrow polydispersity (+/- 1µm).
Note that the particle size is smaller than the droplets as they shrink during the polymerization process. We generated particles of 83 µm diameter, but by varying the flow rates of both continuous and disperse phases, it is possible to obtain different particle sizes.
Conclusion
The fabrication of core–shell polymer microparticles is of great importance due to their wide range of industrial applications, such as in the food industry, cosmetics, and drug delivery. In this short application note, we presented a reproducible solution for UV-crosslinking of micro-sized particles that includes inline droplet generation, spacing, and polymerization.
The system allows for synthesizing particles with narrow polydispersity, tackling a limitation generally encountered in traditional methods for microparticle production.
References
1. Saralidze, K., Koole, L. H. & Knetsch, M. L. W. Polymeric microspheres for medical applications. Materials (Basel). 3, 3537–3564 (2010).
2. Li, W. et al. Microfluidic fabrication of microparticles for biomedical applications. 47, 5646–5683 (2019).
Expertises & Resources
-
Expertise Addressing Air Bubble Issues in Microfluidic Systems Read more
-
Microfluidics White Papers Droplet-based Microfluidics Read more
-
Microfluidic Application Notes UV-Crosslinking of Microcapsules Read more
-
Expert Reviews: Basics of Microfluidics Microfluidic Droplet Production Method Read more