Real-Time Monitoring Platform for Ocular Drug Delivery, Integrating Fluigent’s Flow EZ
Real-time measurement is vital for modern dissolution testing to enhance ocular drug delivery characterization and high-quality control. In this paper published in Pharmaceutics (2023), a real-time monitoring platform, consisting of a microfluidic system, an eye movement platform with temperature sensors, and a concentration probe setup, was developed alongside the PK-Eye™ in vitro model of the human eye. Parallel microfluidic control of PK-Eye™ models demonstrated scalability and reproducibility, achieving physiological intraocular pressure ranges. It showcased the potential for real-time monitoring of pharmaceutical models in preclinical ocular formulation testing.
A University College London (UCL) Paper
Paper: Awwad, S.; Ibeanu, N.; Liu, T.; Velentza-Almpani, A.; Chouhan, N.; Vlatakis, S.; Khaw, P. T.; Brocchini, S.; Bouremel, Y. Real-Time Monitoring Platform for Ocular Drug Delivery. Pharmaceutics 2023, 15 (5), 1444. https://doi.org/10.3390/pharmaceutics15051444
Part of the Faculty of Brain Sciences, the UCL Institute of Ophthalmology holds the top global ranking for ophthalmology studies (per CWUR Rankings by Subject 2017). Collaborating with Moorfields Eye Hospital, they form the largest co-located hub for eye research, education, and care worldwide. Through groundbreaking research in ophthalmology and eye health, their interdisciplinary approach unites scientists, clinicians, and patients, leading to tangible improvements in people’s lives.
In collaboration with UCL, Optceutics Ltd. manufactures advanced in vitro models of the human eye (PK-Eye™) that accelerate the development of longer-acting intraocular medicines. Optceutics Ltd. offers fee-for-service research and strategic collaborations, specializing in preclinical ophthalmic formulation optimization.
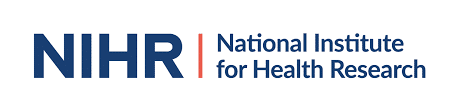
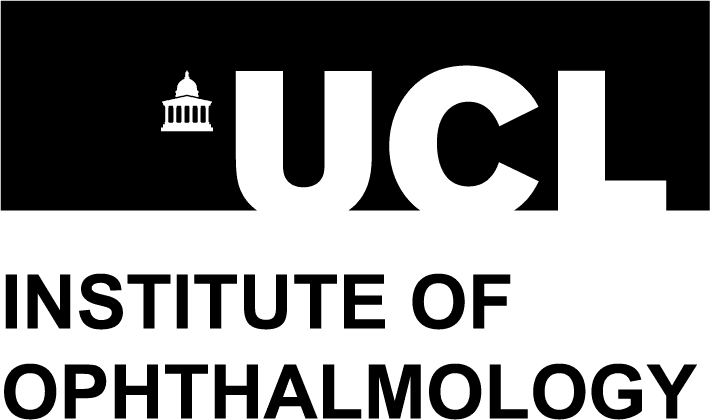
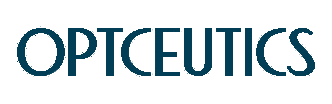
Challenges in Treating Posterior Eye Diseases
The posterior segment of the eye, where diseases like age-related macular degeneration, glaucoma, and diabetic retinopathy occur, poses significant challenges for treatment due to its complexity. These ocular diseases, which can lead to blindness, affect millions worldwide and are becoming more prevailing with the increase in the aging population.[1] To treat them, therapeutic antibodies and proteins have transformed the management of such conditions, often requiring direct injections into the eye for effective ocular drug delivery (figure 1). However, frequent intravitreal injections are burdensome for patients and healthcare systems, prompting the need for improved drug formulations to prolong efficacy and reduce risks. [2,3] Therefore, formulation strategies are essential to address these challenges, necessitating either progress preclinical evaluation in animal models or in vitro models.
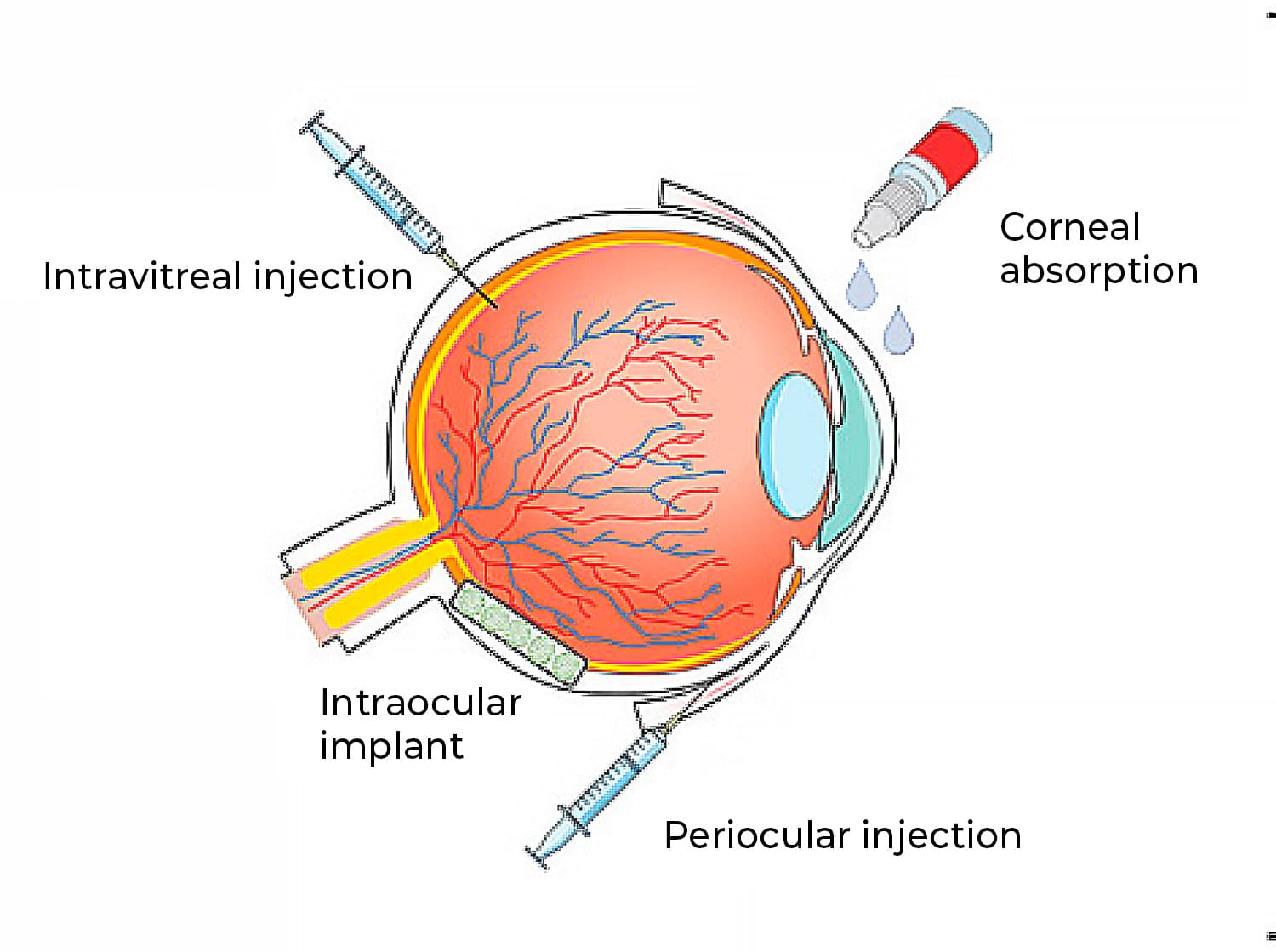
Figure 1: Ocular routes for drug delivery.[4]
How Can in Vitro Models Overcome Limitations in Ocular Drug Delivery Compared to Animal Models?
Classical in-animal models present several limitations in research and drug discovery, including anatomical differences between animal and human eyes. In addition, the formation of anti-drug antibodies complicates the testing of biologics. Ocular tolerability and high costs associated with animal models further highlight the need for alternative testing techniques.[5]
In vitro models offer a promising solution, serving as alternatives or supplements to animal testing during preclinical development. These models not only reduce reliance on animal studies but also help validate dissolution specifications and demonstrate the bioequivalence of active pharmaceutical ingredients. They have been designed for various aims such as assessing protein stability in simulated vitreous fluids, understanding the impact of eye movements, and evaluating drug release/clearance times (figure 2). [6]
Despite significant progress in in vitro ocular formulation testing for posterior eye diseases, there is currently no approved model specifically designed to assess intraocular pharmacokinetics and still lack adequate representation of eye dynamics, such as flow rate and compartmentalization, crucial for understanding drug kinetics and stability within the eye.
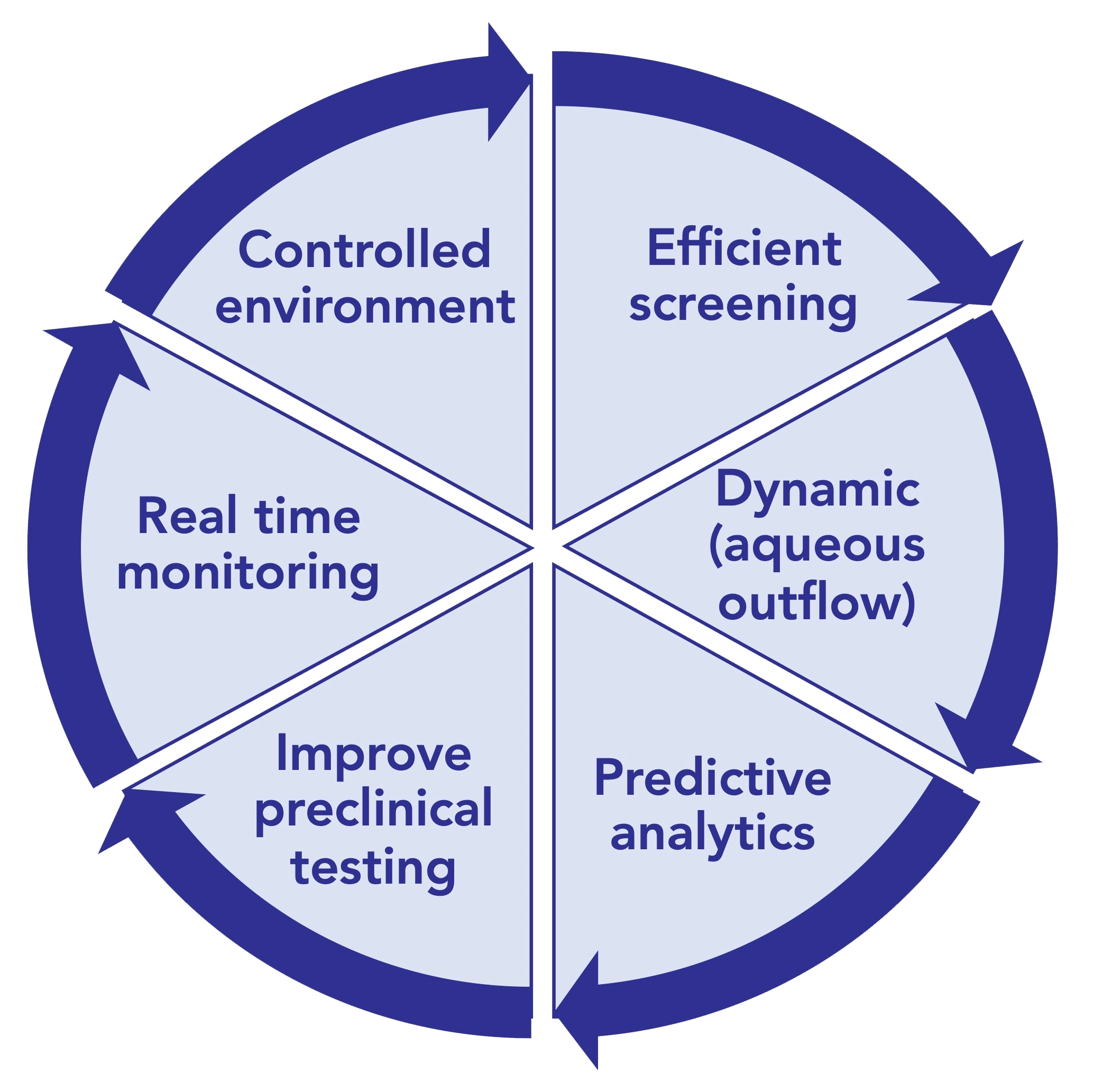
Figure 2: Ultimate goals for In-vitro eye models to evaluate ocular drug delivery.[7]
PK-Eye™ Model: Example of Real-Time Monitoring Platform for Ocular Drug Development.
The PK-Eye™ model, designed and manufactured by Optceutics Ltd., emerged as a robust and user-friendly tool, addressing the limitations of in vivo models for developing long-acting intraocular medicines. Combining this in vitro model with real-time monitoring systems will facilitate more efficient preclinical testing by increasing data collection while minimizing manual intervention.[8]
In a paper published in Pharmaceutics (2023), a newly designed real-time monitoring platform was scaled and automated for ocular drug delivery. This platform aims to provide proof-of-concept for a fully automated and optimized PK-Eye™ model testing setup, to accelerate intraocular drug development by streamlining sample analysis and improving accuracy (figure 3).
Key features of the platform included monitoring flow, temperature, eye movements, and concentration evaluation of labeled protein molecules (figure 4).
How to Scale up Real-Time Monitoring
Each PK-Eye™ model was connected to a FlowEZ microfluidic controller, with buffer (PBS, pH 7.4). These controllers were linked to an FLPG plus 2-bar pressure source. The pressure and flow were managed via the Fluigent A-i-O program (previous version of Oxygen). Scalability was tested with configurations running 1× LineUp Flow EZ controlling 1–6 models (figure 5). Experiments ran at a fixed flow rate of 2 μL/min at 37°C, with a 48-hour equilibration period before data recording. The flow rates were recorded with flow units S.
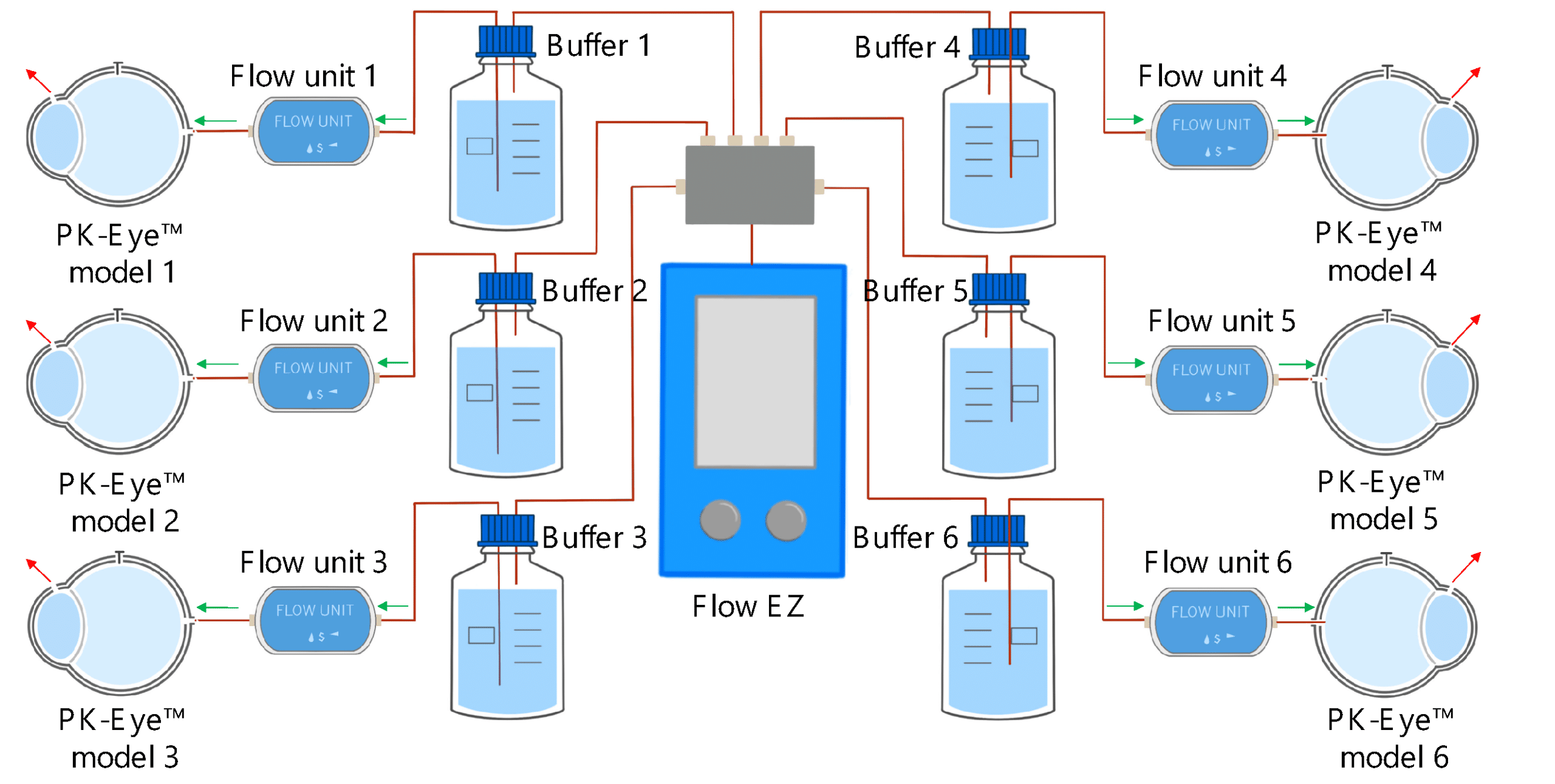
Figure 5: Experimental setup of flow rate control with 1:6 ratios using PK Eye models in PBS at RT with a pressure control of for 24h. [7]
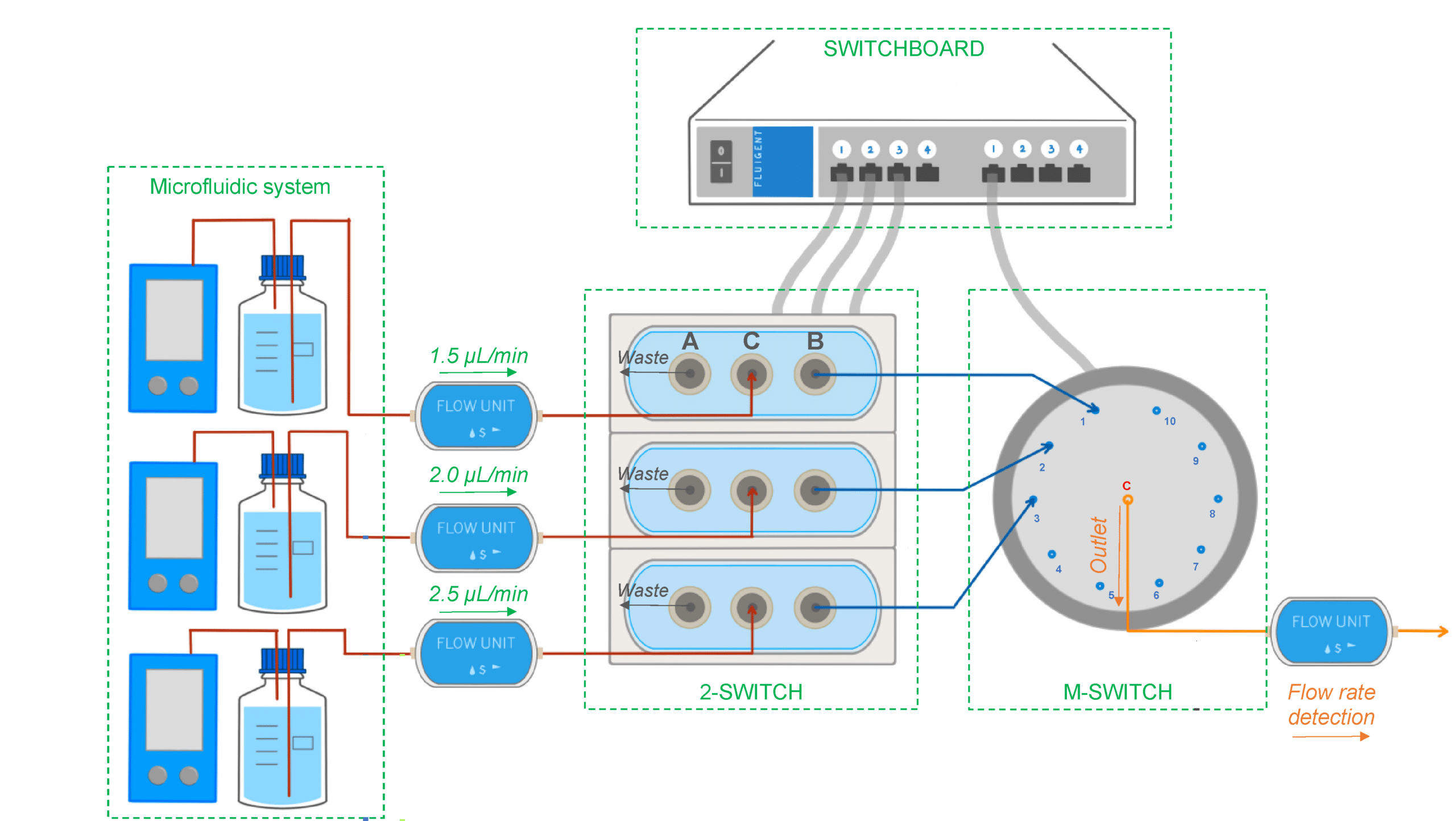
Figure 6: Experimental setup used to select different flow lines to be analyzed by the concentration detection unit to monitor multiple PK-Eye™ models.[7]
To enable real-time monitoring of drug release profiles across multiple models or fluid inlets, microfluidic valves from Fluigent were integrated into the system. It included three 3-port/2-way microfluidic valves (2-SWITCH), an 11-port/10-position rotary valve (M-SWITCH), and a SWITCHBOARD (Previous version of SWITCH EZ) (figure 6). This platform facilitated the selective collection of outflows from different models or inlets into the concentration detector, allowing for varied release profile readouts.
Next, the integration of this platform with a concentration probe was demonstrated. A PK-Eye™ model with a membrane was connected to the 2-SWITCH, allowing switching between fluid outlets. PBS buffer was pumped through the model while the outflow was alternated between the model and a PBS reservoir. Alexa albumin was injected into the model, and the system toggled between the model and reservoir every 2 hours for real-time detection by the concentration probe.
Proof-of-Concept: Unlock Stability and Scalability Using Flow Controllers vs Peristaltic Pumps
Traditional syringe or peristaltic pumps are commonly used in pharmaceutical labs to impose a constant flow rate, but they often result in cyclical flow rate variations. Fluigent’s pressure-driven controller (FlowEZ) was introduced in this work to allow large-scale experiments, offering smooth flow rates with minimal variation and rapid response to pressure or flow rate changes. Troubleshooting was also easier with this system compared to peristaltic pumps (table 1).
Table 1: Comparison between the PK-Eye platform based on a peristaltic pump system or a flow controller system.
Model-Platform | Flow based on peristaltic pump (1st Generation PK-Eye™) | Flow based on pressure-driven controllers (Latest PK-Eye™ model) |
---|---|---|
Pressure-flow Monitoring | No | Yes (with graph readout) |
Simultaneous models testing (n) | 8 | 48 |
Circadian rhythm | No | Yes (with graph readout) |
Simulated vitreous fluids leakage monitoring | No | Yes (with graph readout) |
Eye movement monitoring | No | Yes, eye movements shown (with graph readout) |
Temperature monitoring | Thermometer | Temperature sensors |
Concentration readout | Manual sampling and HPLC analysis | Manual sampling and HPLC analysis, and use of concentration probe setup |
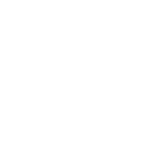
“A pressure-controlled flow system, as opposed to syringe pumps or peristaltic pumps, was introduced to allow large scale experiments by allowing a single pressure source to control several models simultaneously. The flow rate controlled by compressed air results in an extremely smooth flow rate with quasi-null flow rate variation”
S.Awwad et al., Pharmaceutics 2023,15, 1444.
In parallel systems, pressure remained constant, allowing multiple models to be controlled simultaneously from a single pressure source with the ability to run up to six models concurrently. Ratio experiments demonstrated this, with each model flowing within a natural aqueous humor flow rate range (between 1.5 µL/min and 2.7 µL/min), highlighting the uniformity of the setup (figure 7).
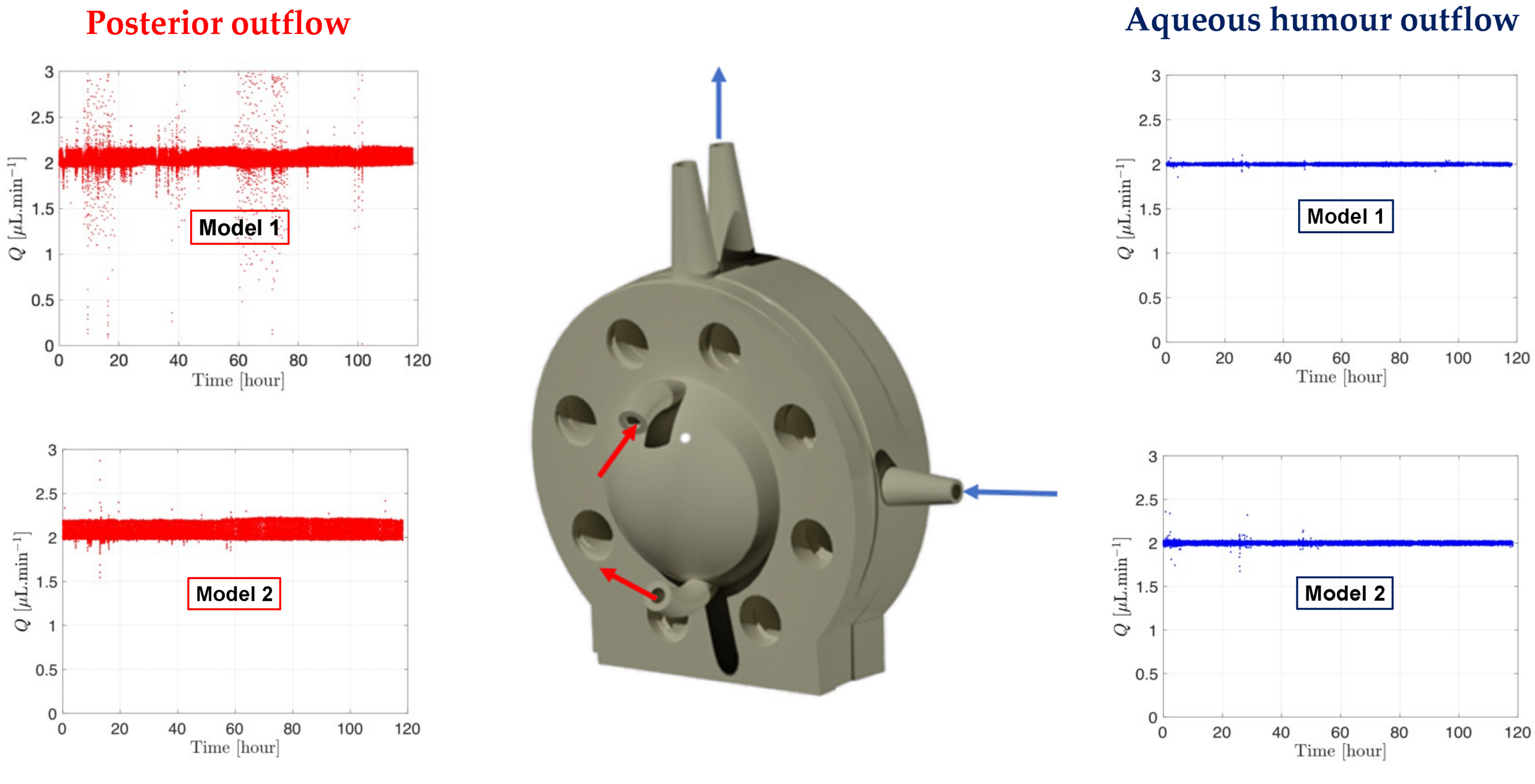
Figure 8: Graph readout from the microfluidic system showing the flow and pressure from the models with SVF at 37°C. [7]
The microfluidic setup with the PK-Eye™ model ensured consistency and detects simulated vitreous fluids (SVF) leakage during testing, critical for maintaining quality control. Stability tests with the model showed consistent pressure and flow rates over 5 days, indicating successful SVF containment without leakage (figure 8). This highlights the robustness of both the microfluidic system and model setup throughout the experiment.
The integration of the 2-SWITCH/M-SWITCH setup facilitated continuous drug quantification across multiple model post-clearance from the PK-Eye™ model, utilizing a connected concentration probe through the M-SWITCH. The platform effectively selected different fluid outlets automatically from this combination of M-SWITCH and 2-SWITCH, enabling real-time monitoring of drug release between multiple flow outlets (figure 9).
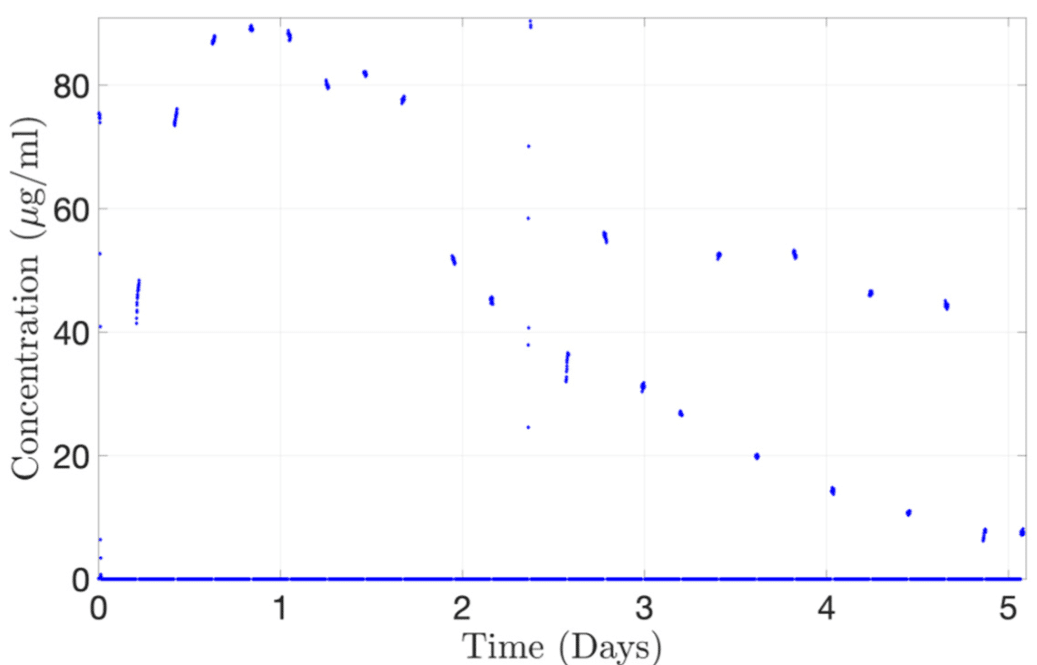
Figure 10: Continuous monitoring of Alexa albumin from the concentration probe connected to 2-SWITCH/M-SWITCH platform.[7]
As a proof-of-concept, the concentration-time profile of Alexa albumin injected into the PK-Eye™ was determined using a concentration probe setup. The platform successfully reproduced the drug release curve, reaching a maximum concentration of approximately 89 μg/mL at 20 hours. This demonstrates the automatic selection of channels flowing through the concentration probe (figure 10). Thus, it highlights the potential for automated redirection of different flows to record the concentration of cleared drugs, showcasing the real-time monitoring capabilities of the platform for ocular drug delivery.
Conclusion
The PK-Eye™ model, developed by Optceutics Ltd., fast-tracks intraocular drug development through real-time monitoring on an automated platform. This platform records pressure, flow, temperature, eye movement, and concentration in connected models, helping in ocular drug delivery studies. Computer-controlled microfluidics mimic ocular flow dynamics with high stability and responsiveness, while an eye movement platform studies their impact on drug clearance. The 2-SWITCH/M-SWITCH platform expands monitoring capacity for multiple models simultaneously.
Related products
Expertise & Resources
-
Microfluidics Article Reviews Microfluidic technology for engineered nanoparticles in nanomedicine Read more
-
Expert Reviews: Basics of Microfluidics Microfluidics for vaccine development Read more
-
Expert Reviews: Basics of Microfluidics Flow control for droplet generation using syringe pumps and pressure-based flow controllers Read more
References:
(1) Ranta, V.; Urtti, A. Transscleral drug delivery to the posterior eye: Prospects of pharmacokinetic modeling. Adv. Drug Deliv. Rev. 2006, 58, 1164–1181.
(2) Jager, R.D.; Aiello, L.P.; Patel, S.C.; Cunningham, E.T. Risks of intravitreous injection: A comprehensive review. Retina 2004, 24, 676–698.
(3) Thrimawithana, T.; Young, S.; Bunt, C. Drug delivery to the posterior segment of the eye. Drug Discov. Today 2011, 16, 270–277.
(4) Souto, E. B.; Dias-Ferreira, J.; López-Machado, A.; Ettcheto, M.; Cano, A.; Camins Espuny, A.; Espina, M.; Garcia, M. L.; Sánchez-López, E. Advanced Formulation Approaches for Ocular Drug Delivery: State-Of-The-Art and Recent Patents. Pharmaceutics 2019, 11 (9), 460.
(5) Laude, A.; Tan, L.E.;Wilson, C.G.; Lascaratos, G.; Elashry, M.; Aslam, T.; Patton, N.; Dhillon, B. Intravitreal therapy for neovascular age-related macular degeneration and inter-individual variations in vitreous pharmacokinetics. Prog. Retin. Eye Res. 2010, 29, 466–475.
(6) Awwad, S.; Henein, C.; Ibeanu, N.; Khaw, P.T.; Brocchini, S. Preclinical challenges for developing long acting intravitreal medicines. Eur. J. Pharm. Biopharm. 2020, 153, 130–149.
(7) Awwad, S.; Ibeanu, N.; Liu, T.; Velentza-Almpani, A.; Chouhan, N.; Vlatakis, S.; Khaw, P. T.; Brocchini, S.; Bouremel, Y. Real-Time Monitoring Platform for Ocular Drug Delivery. Pharmaceutics 2023, 15 (5), 1444.
(8) Liu, T.; Ibeanu, N.; Brocchini, S.; Khaw, P. T.; Bouremel, Y.; Awwad, S. Development of an in Vitro Model to Estimate Mass Transfer from the Anterior Cavity. Front. Drug. Deliv. 2022, 2, 1025029.