Microfluidic Chitosan Microcapsules Production
Microcapsules with a chitosan shell and an oily core have been extensively researched in recent years due to their biocompatibility and non-toxic biopolymer. In this application note, chitosan-shell/oily-core microcapsules are generated using the Raydrop double emulsion- developed and manufactured by Secoya and Fluigent pressure-based flow controllers. The influence of the fluidic parameters on the size and the release from the oil across the shell are studied and presented.
Secoya developed and manufactured the RayDrop used to perform this application note.
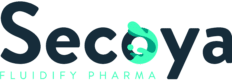
Introduction to Chitosan Microcapsules
Why is droplet microfluidics preferred for microcapsule production?
In recent decades, core-shell microcapsules have become integral in the pharmaceutical, cosmetic, and food industries for material delivery and release. Microencapsulation technologies play a crucial role in safeguarding enclosed materials and ensuring prolonged efficacy by controlling the release rate.
Traditional bulk microencapsulation methods, relying on intense mixing of immiscible solutions, often involve intricate processes and equipment. These methods face limitations in controlling microcapsule sizes and achieving monodispersity. In contrast, microfluidics offers a superior approach, allowing for the production of monodisperse double emulsions with precise control over both size and structure.
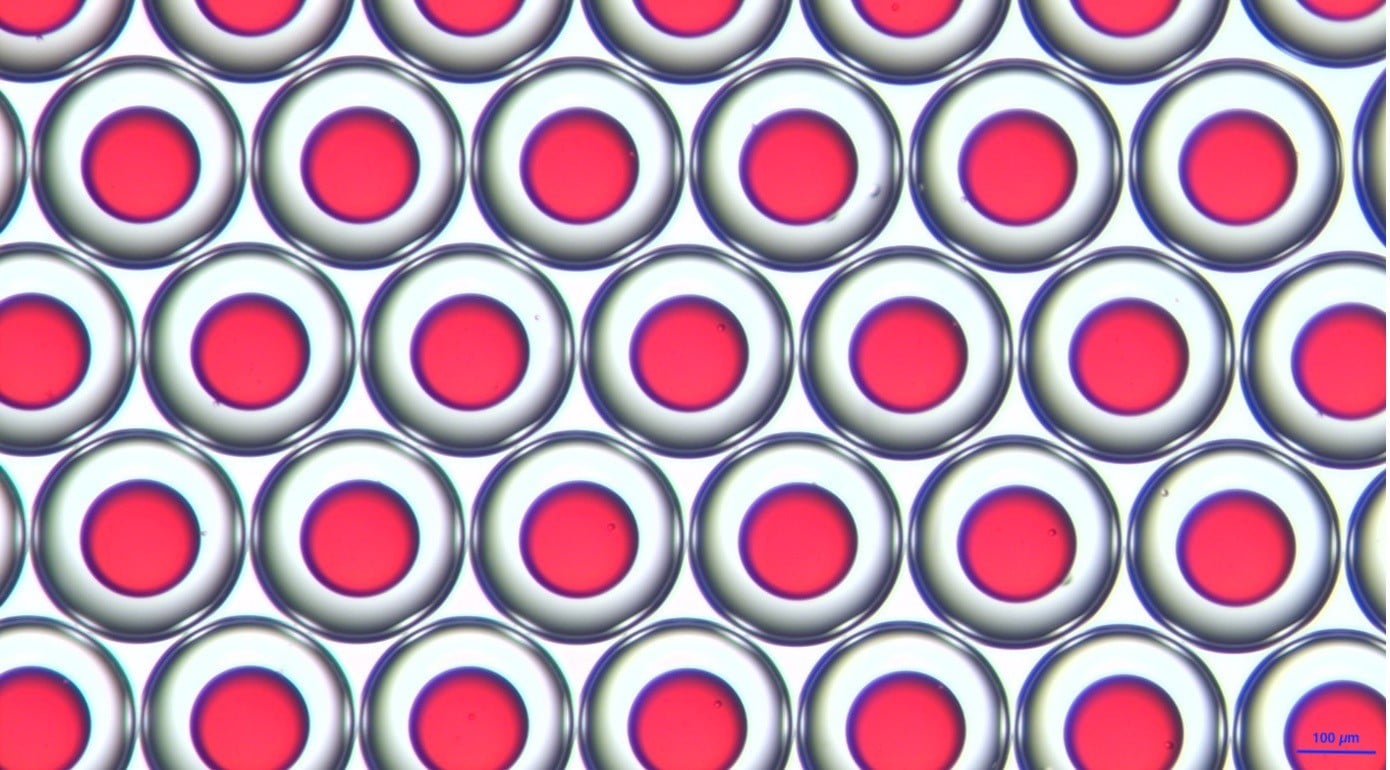
Why is chitosan an interesting encapsulating agent?
Chitosan is a polysaccharide derived from chitine and most commonly obtained from the exoskeleton of crustaceans and from fungi. It has always been considered as a promising encapsulating agent for all kinds of applications due to its biocompatibility, lack of toxicity, antibacterial activity, high availability, and low cost. It is nature’s most important organic compound after cellulose. Also, chitosan has unique chemical properties due to its cationic charge in solution.
Chitosan microcapsules have been widely used as an encapsulating agent for several applications, such as food processing, biomedical and pharmaceutical, wastewater treatments, and textiles, alone or in combination with other polysaccharides or proteins to improve the shell properties. The application of chitosan microcapsules in textiles follows the current interest of industries in functionalization technologies that give different properties to products, such as aroma finish, insect repellent, antimicrobial activity, and thermal comfort.
How to generate chistosan-shelled double emulsions
Double emulsion platform
We performed chitosan microcapsule generation with the Complex Emulsions Production Platform, a lab system integrating all the components needed to produce simple and double emulsions.
Figure 3: Experimental set-up to produce double emulsion
Material for chitosan bead generation
Reagents
Core phase:
Soybean oil (8001-22-7, Sigma-Aldrich) containing red dye Sudan IV (Sigma-Aldrich)
Shell phase:
Water containing 2% chitosan (viscosity 30-100 mPa·s, Glentham Life Sciences UK), 2% acetic acid (Sigma-Aldrich), 1% Pluronic® F-127 (Sigma-Aldrich)
Continuous phase:
1-octanol (Glentham Life Sciences UK) containing 2% Span 80 (Sigma-Aldrich)
Collection phase:
Heptane (VWR) containing 2% Span 80 (Sigma-Aldrich) and 0,3 wt% glutaraldehyde (50% in H2O, Glentham Life Sciences UK)
The pressure controllers used are 7 bar full scale. The maximum pressure used for the generation of double emulsions with large shells is 2440 mbar (corresponding to a shell phase flow rate of 24.3 µL/min). Though in this scenario, maximum working pressure is 1650 mbar. Priming and cleaning steps can require a pressure higher than 2 bar.
Synthesis of chitosan-shelled double emulsions
Monodisperse chitosan microcapsules synthesis is performed in 2 main steps:
- Generation of monodisperse double emulsion in the Raydrop
- Capsule formation by reticulation of the chitosan shell in the collection bath
1. Double Emulsion Generation
To generate droplets, the system must first be primed with pure solvent in the shell phase (here water + 2% acetic acid). Once droplet formation is stable, the shell phase is switched to the chitosan-based solution. This avoids clogging issues during the transient phase.
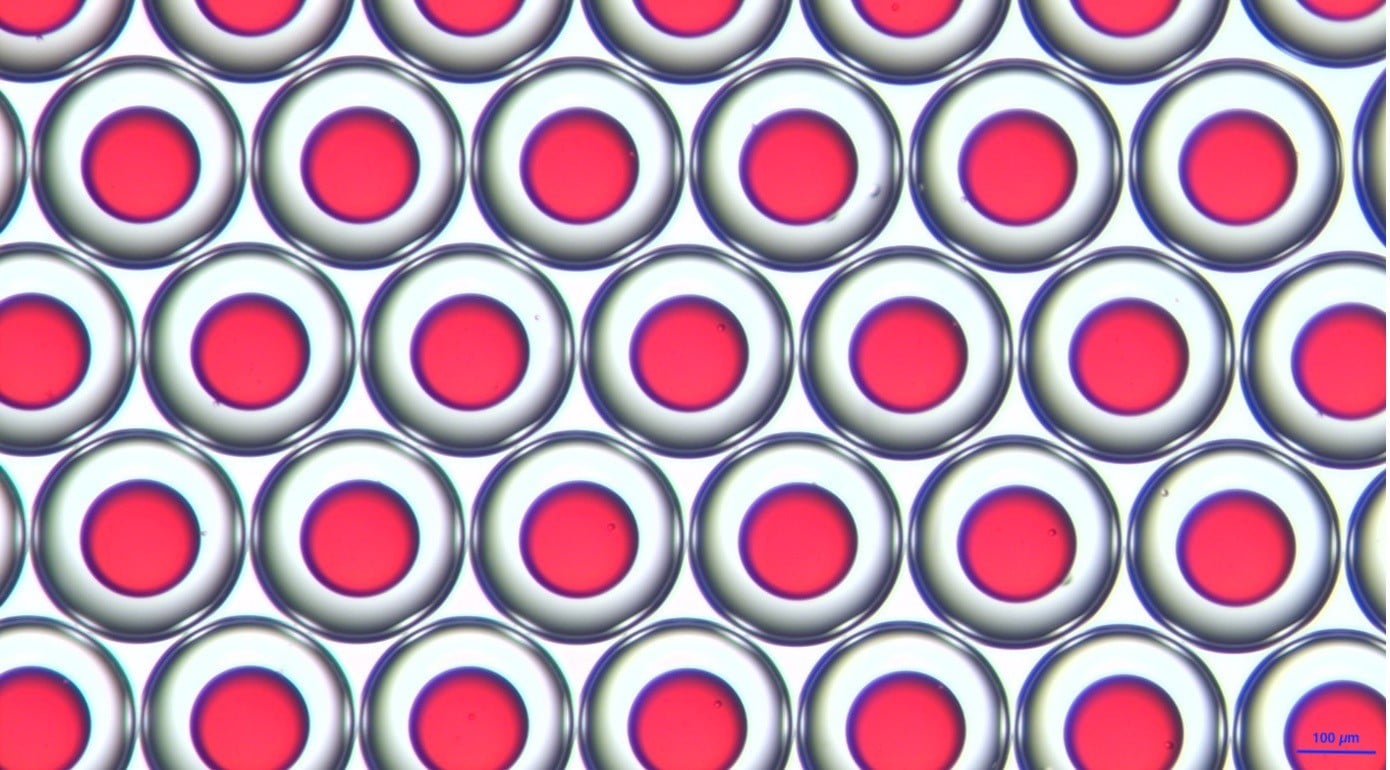
2. Chitosan Microcapsules Formation
After generation, the droplets are collected in a cross-linking solution of 0.3% glutaraldehyde in hexane. The chitosan reacts with glutaraldehyde by solvent extraction and chemical cross-linking based on the Schiff base reaction. The droplets are solidified and become glutaraldehyde cross-linked chitosan microcapsules.
Figure 6: Glutaraldehyde cross-linked chitosan microcapsules on the cross-linking bath. On the left, after 4 minutes in the cross-linking bath. On the right, after 1h in the cross-linking bath. The shell thickness decreases and becomes progressively yellow, as a part of its water content diffuses in the continuous phase. Expelled water is clearly visible wetting the capsules.
Results: production of stable, monodisperse microcapsules
In this application note, different parameters were studied. First, the evolution of the droplets over time was observed. Then, the influence of the middle and the outer phase flow rates were studied.
Evolution of the Droplet Diameter During the Cross-Linking Process
After generation, the double emulsion droplets are collected into the collection solution. For a given sample, several measurements of the capsule diameter are done at different times. The evolution of the diameter is highlighted in Figure 7.
Influence of the Middle Phase Flow Rate
After analyzing the size of the chitosan microcapsules over time, the influence of the middle phase flow rate is observed. We varied the shell flow rate at fixed continuous and core phase flow rates. The evolution of these two diameters is underlined in Figure 8. Figure 9 shows the evolution of the thickness of the droplet with the evolution of the shell flow rate.
Influence of the Outer Phase Flow Rate
Here, the shell flow rate and core flow rate are fixed but the continuous phase flow rate is varying. The change in diameter as a function of flow rate is shown in Figure 10.
Conclusion
The production of stable, monodispersed microcapsules with a solid chitosan shell and a liquid oil, non-polar core using a microfluidic system has been successfully achieved. The Fluigent microfluidic platform also allows one to tune the core diameter and the shell thickness by adjusting the flow rates of the different fluids. Due to excellent oil encapsulation properties and a very limited leakage over time, these microcapsules can be used in a wide range of applications, including the encapsulation of volatile products like mint oil [3] as well as specific drugs, which will be delivered according to the pH acidity [2].
References
[1] KILDEEVA, N. R., PERMINOV, P. A., VLADIMIROV, L. V., NOVIKOV, V. V. and MIKHAILOV, S. N., 2009. About mechanism of chitosan cross-linking with glutaraldehyde. Russian Journal of Bioorganic Chemistry. 1 May 2009. Vol. 35, no. 3, p. 360–369. DOI 10.1134/S106816200903011X.
[2] LIU, Li, YANG, Jian-Ping, JU, Xiao-Jie, XIE, Rui, LIU, Ying-Mei, WANG, Wei, ZHANG, Jin-Jin, NIU, Catherine Hui and CHU, Liang-Yin, 2011. Monodisperse core-shell chitosan microcapsules for pH-responsive burst release of hydrophobic drugs. Soft Matter. 3 May 2011. Vol. 7, no. 10, p. 4821–4827. DOI 10.1039/C0SM01393E.
[3] DU, Yuhan, MO, Liangji, WANG, Xiaoda, WANG, Hongxing, GE, Xue-hui and QIU, Ting, 2020. Preparation of mint oil microcapsules by microfluidics with high efficiency and controllability in release properties. Microfluidics and Nanofluidics. June 2020. Vol. 24, no. 6, p. 42. DOI 10.1007/s10404-020-02346-2.
Related Resources
- Microfluidic Application Notes
Alginate Microcapsule Synthesis
Discover - Microfluidic Application Notes
Agarose Microcapsules Synthesis
Discover - Microfluidic Application Notes
PLGA microcapsules synthesis
Discover - Microfluidics White Papers
Double emulsion for the generation of microcapsules – a Review
Discover - Microfluidic Application Notes
Double Emulsion Generation
Discover