Microfluidic Transistor for Precise Fluid Control
Just as electronic transistors revolutionized circuit design, microfluidics has transformed fields like biology and diagnostics. However, replicating transistor-like precision in fluid control has remained a challenge. This study introduces a microfluidic transistor that mimics electronic transistor behavior, overcoming past limitations.
Using the Fluigent’s Pressure Controller to regulate the system, this microfluidic transistor was employed in fluidic circuits such as amplifiers and particle dispensers, advancing lab-on-a-chip automation with precise control over liquids and particles.
A Paper from the BioMEMS and Nanoscale Engineering Group
Paper: Gopinathan, K. A.; Mishra, A.; Mutlu, B. R.; Edd, J. F.; Toner, M. A Microfluidic Transistor for Automatic Control of Liquids. Nature 2023, 622 (7984), 735–741
This study is the result of a collaboration between the BioMEMS Resource Center, the Cancer Center, the Department of Surgery, from the Massachusetts General Hospital (Boston, MA, USA) and the Shriners Children’s (Boston, MA, USA). Led by Dr. Mehmet Toner, the BioMEMS and Nanoscale Engineering group develops advanced microfluidic platforms to study cell and tissue behavior. Key projects include continuous-flow devices for real-time detection of cell secretions, analysis of cancer cell motility, and microfluidic systems for isolating circulating tumor cells and advancing diagnostics.

What is a Transistor and its Purpose?
A transistor is a semiconductor device that regulates or controls the flow of electrical current or voltage, amplifies signals, and acts as a switch or gate. It typically consists of three layers or terminals of semiconductor material that can carry current. The transistor was invented in 1947 at Bell Laboratories by John Bardeen, Walter Brattain, and William Shockley, marking a breakthrough that replaced the bulky vacuum tubes used in electronics (Figure 1).
Transistors quickly became crucial in modern electronics, amplifying weak signals and switching between “on” and “off” states to control signal flow.1 They form the foundation of integrated circuits, where large numbers of transistors are interconnected to create microprocessors, memory chips, and other electronic devices. Transistors revolutionized technology by enabling miniaturization, reducing power consumption, and increasing efficiency.
Their invention led to the development of faster, smaller, and more affordable electronics, fueling advancements in computing, telecommunications, and many other industries (automative, energy, healthcare and medical devices).2,3
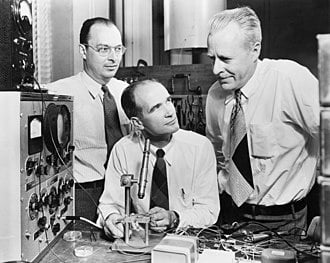
Figure 1: The Bell Lab: William Shockley, Walter Brattain and John Barden in 1948. (*Picture by AT&T. Public domain).
Is Mimicking Transistor Functions Possible in Microfluidics?
Microfluidics and microelectronics share a fundamental analogy in their goal of controlling small-scale flows and signals with high precision. Microfluidic systems aim to manage fluid flow, but unlike electrical signals, fluid dynamics are influenced by viscosity, pressure, and channel geometry. Significant advancements have been made in microfluidic applications such as particle sorting, signal amplification, and cell manipulation, though challenges remain in fully replicating the same level of efficiency and precision seen in electronic systems.
For instance, while resistance in electronic circuits is well-understood, fluidic resistance is more complex, depending on factors like flow dynamics and microchannel design (Figure 2).
Additionally, electronic transistors exhibit essential functions such as saturation, proportional amplification, and reliable on/off switching, which have not yet been fully replicated in microfluidic systems.4–7 Achieving transistor-like amplification in microfluidics could enhance precision, speed, and reliability, enabling the use of electronic design strategies in biological and chemical processing for lab-on-a-chip and automated diagnostics.8,9
Aim of the Study
This case study presents, for the first time, a microfluidic transistor that replicates all functions of an electronic transistor, including amplification, saturation, and switching, based on flow limitation. It enables fluidic versions of amplifiers, regulators, NAND gates, and more, allowing on-chip signal processing.4
As proof of concept, the fluidic transistor is used in an autonomous particle dispenser that detects and manipulates individual particles, demonstrating its potential for fully autonomous lab-on-a-chip systems.
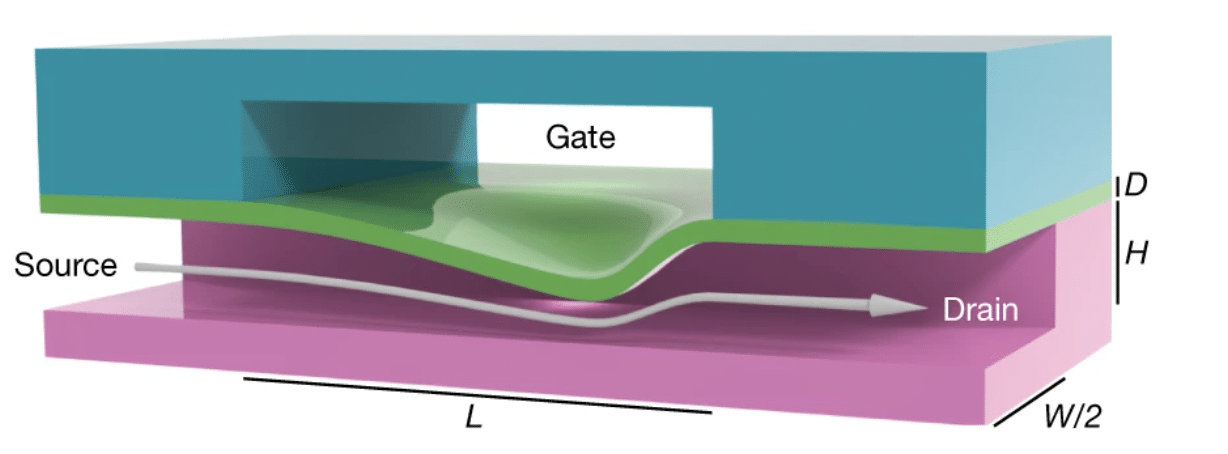
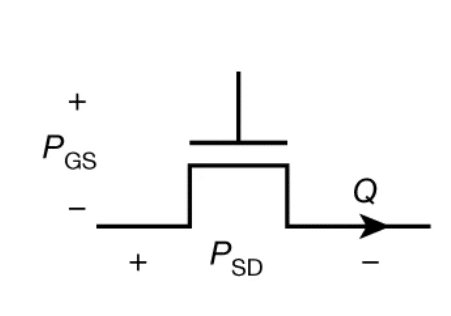
Figure 3: The developed microfluidic transistor and its schematic symbol.4
Methodology: How to Include a Microfluidic Transistor in Your System
The microfluidic transistor is constructed using elastomer and soft-lithography techniques, consisting of two crossed liquid channels separated by a deformable membrane. When a pressure difference is applied between the source and drain terminals, the membrane deforms, creating flow limitation, which is key to the transistor’s amplification function. This flow limitation can be modulated by applying pressure between the gate and source terminals.
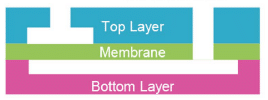
The transistor was characterized by using principles similar to those of a p-channel junction field-effect transistor, demonstrating fluidic analogues to key electronic circuit elements such as amplifiers, level shifters, and latches.
Fluigent’s LineUp Flow-EZ was used for precise pressure control during testing. The Fluigent Software Development Kit (SDK) was employed for integration and automation of the fluidic system, ensuring efficient control over the entire experimental process. Fluidic connections were made using FEP tubing and PEEK fittings, and the flow measurements were analyzed with MATLAB to evaluate the transistor’s performance in microfluidic systems.
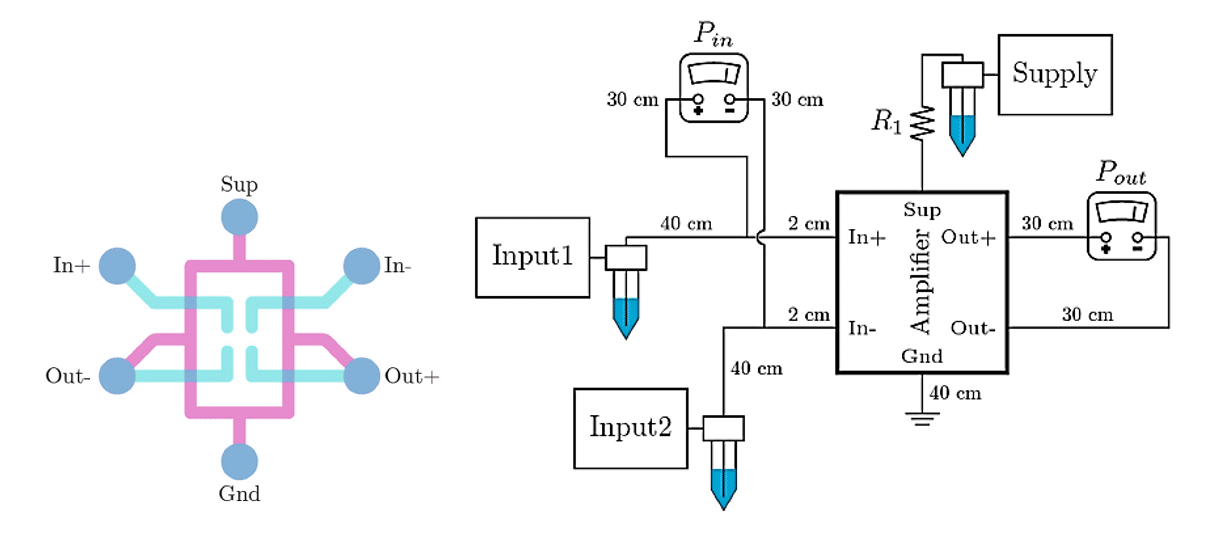
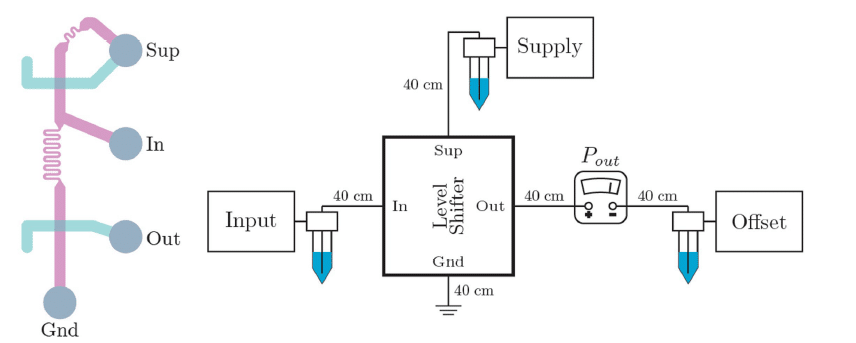
The microfluidic transistor was utilized in a smart particle dispenser system. The device consisted of a particle trap that detects and manipulates particles using pressure-controlled fluidic circuits. A suspension of 40-μm-diameter polystyrene microspheres in PBS was used for testing, achieving a concentration of approximately 30 beads per milliliter.
When a particle is trapped, a slight pressure increase upstream of the trap is detected and amplified by the amplifier circuit block. This change is compared with a reference threshold pressure, producing complementary signals indicating the particle’s presence. A latch circuit ensures the complementarity of the signals while suppressing noise, and level shifters adjust the output signals for control.
Proof-of-Concept: A Microfluidic Transistor for Complete Liquid Control Functions
The key functional characteristic of the microfluidic transistor is its ability to achieve proportional amplification of fluidic signals, analogous to its electronic counterpart. This amplification is quantified by the intrinsic gain, which is derived from the applied source-drain (PSD) and gate-source (PGS) pressures. A large operating region, where the intrinsic gain exceeds one, enables the transistor to perform efficient signal amplification (Figure 7). This is achieved through flow limitation, a phenomenon where the flow rate remains largely unaffected by increases in pressure beyond a specific threshold, mirroring the saturation behavior observed in field-effect transistors.
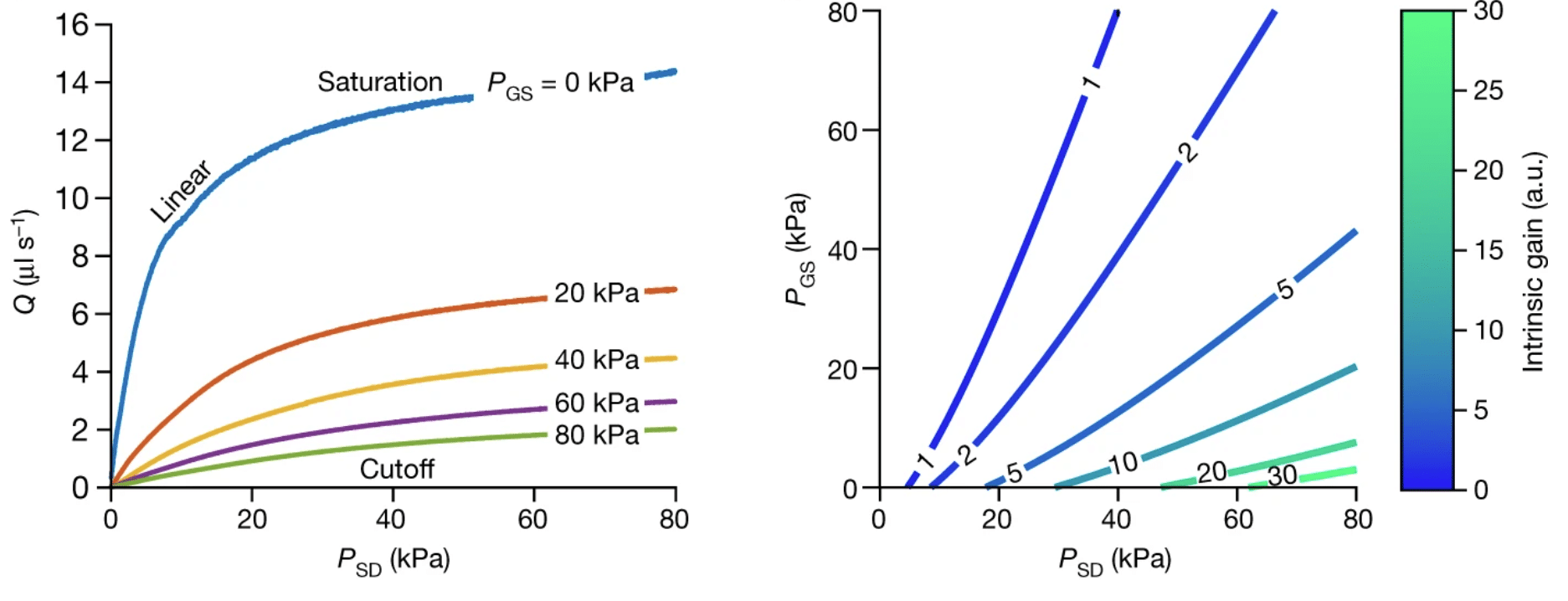
To demonstrate the versatility of the microfluidic transistor, several electronic circuit elements were replicated in the fluidic domain. The differential amplifier, operating in a common-source topology, increases input differential pressure signals with a gain exceeding 20 (Figure 8). The level shifter circuit, based on the common-drain topology, shifts the baseline of input signals to higher levels while maintaining their signal morphology, enabling modular circuit design with varying biasing pressures (Figure 9). The SR latch (Set-Reset), a bistable multivibrator, stores fluidic states by transitioning between two stable output states, thereby acting as a memory element for sequential operations (Figure 10).
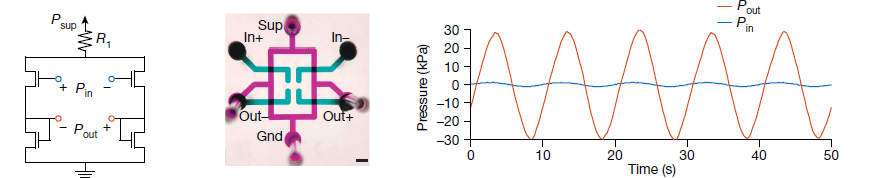
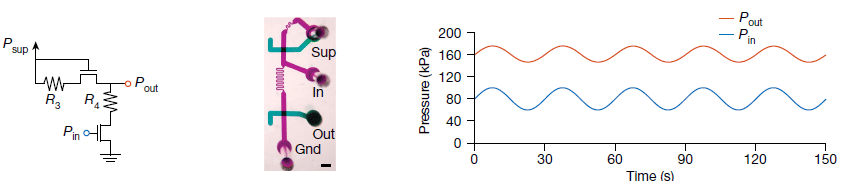
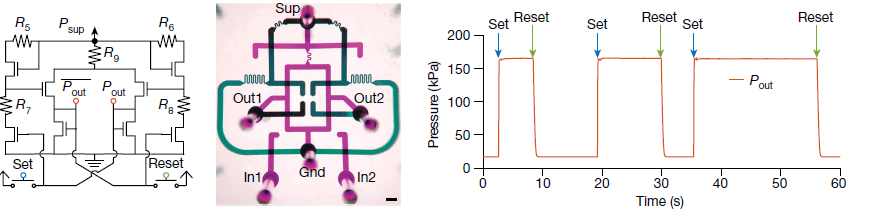
The fluidic transistor was applied to a particle dispensing system, where it enabled the concentration and ordering of particles in a fluidic stream. By employing a feedback loop between the “Sense” and “Trig” lines, the system achieves deterministic particle ordering, as evidenced by a reduction in particle spacing mean and standard deviation. This application demonstrates the potential of microfluidic transistors to autonomously control the manipulation of physical samples, showcasing their capability for integration into lab-on-a-chip devices for complex fluidic processing.
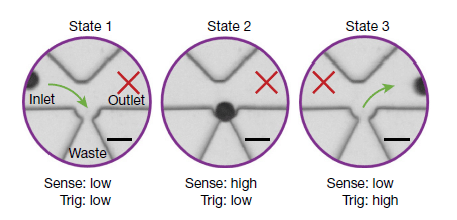
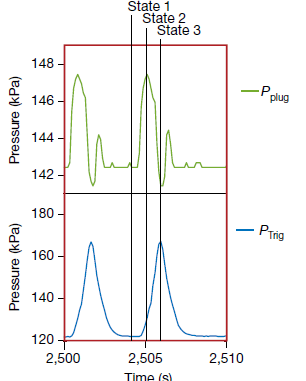
Figure 11: (Left) Overview of the smart dispenser operation, showing the core microfluidic trap in various states as it senses and dispenses a single particle (scale bars, 50 μm). (Right) A representative dispense event to observe the three states in function of pressure.4
Conclusion
In this case study, researchers from the BioMEMS and Nanoscale Engineering Group (Massachusetts General Hospital) present a microfluidic transistor that achieves proportional amplification and replicates all key transistor topologies. This enables the creation of fluidic circuits based on electronic analog and digital designs, functioning autonomously. With the ability to process fluidic signals and control individual particles, this technology offers significant potential for advancing lab-on-a-chip systems and automatic control in microfluidic applications.
Expertises and resources
-
Microfluidics White Papers An exploration of Microfluidic technology and fluid handling Read more
-
Microfluidics case studies The Hebrew University: Encapsulation and culture in 3D hydrogels for Single cell sequencing Read more
-
Microfluidics case studies University of Maryland: Microfluidic System for Robotic that can Play Nintendo Read more
References
1. Iwai, H. & Misra, D. The Transistor was Invented 75 Years Ago: A Big Milestone in Human History. Electrochem. Soc. Interface 31, 65–72 (2022).
2. The lost history of the transistor. IEEE Spectr. 41, 44–49 (2004).
3. History of Semiconductor Engineering. (Springer Berlin Heidelberg, Berlin, Heidelberg, 2007). doi:10.1007/978-3-540-34258-8.
4. Gopinathan, K. A., Mishra, A., Mutlu, B. R., Edd, J. F. & Toner, M. A microfluidic transistor for automatic control of liquids. Nature 622, 735–741 (2023).
5. Duncan, P. N., Nguyen, T. V. & Hui, E. E. Pneumatic oscillator circuits for timing and control of integrated microfluidics. Proc. Natl. Acad. Sci. 110, 18104–18109 (2013).
6. Kim, S., Lai, D., Park, J. Y., Yokokawa, R. & Takayama, S. Microfluidic Automation Using Elastomeric Valves and Droplets: Reducing Reliance on External Controllers. Small 8, 2925–2934 (2012).
7. Mosadegh, B., Bersano-Begey, T., Park, J. Y., Burns, M. A. & Takayama, S. Next-generation integrated microfluidic circuits. Lab. Chip 11, 2813 (2011).
8. Convery, N. & Gadegaard, N. 30 years of microfluidics. Micro Nano Eng. 2, 76–91 (2019).
9. Li, S.-S. & Cheng, C.-M. Analogy among microfluidics, micromechanics, and microelectronics. Lab. Chip 13, 3782 (2013).