A microfluidic Artery-on-a-Chip using Fluigent’s Microfluidic Flow Control System, the MFCS
This study utilizes organ-on-a-chip technology to create an artery-on-a-chip (AoC) model, aiming to mimic the structure of medium-to-large size arteries and their response to hemodynamic forces. The AoCs are exposed to in vivo-like shear stress or maintained in static conditions to identify novel genes affected by shear stress. Patient-derived specimens from both diseased and non-diseased vessel regions are employed to investigate the role of shear stress in diseases like carotid artery disease (CAD) and abdominal aortic aneurysms (AAA). Indeed, the AoC serves as a platform for drug testing, replicating the effects of the therapeutic agent Lenvatinib observed in preclinical AAA studies, thus expanding our understanding of its therapeutic potential in a multicellular context.
Paloschi V, Pauli J, Winski G, Wu Z, Li Z, Botti L, Meucci S, Conti P, Rogowitz F, Glukha N, Hummel N, Busch A, Chernogubova E, Jin H, Sachs N, Eckstein HH, Dueck A, Boon RA, Bausch AR, Maegdefessel L. Utilization of an Artery-on-a-Chip to Unravel Novel Regulators and Therapeutic Targets in Vascular Diseases. Adv Healthc Mater. 2023 Oct 5:e2302907. doi: 10.1002/adhm.202302907.
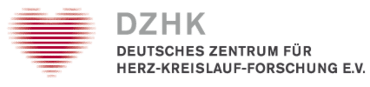
Find more about German Center for Cardiovascular Research DZHK
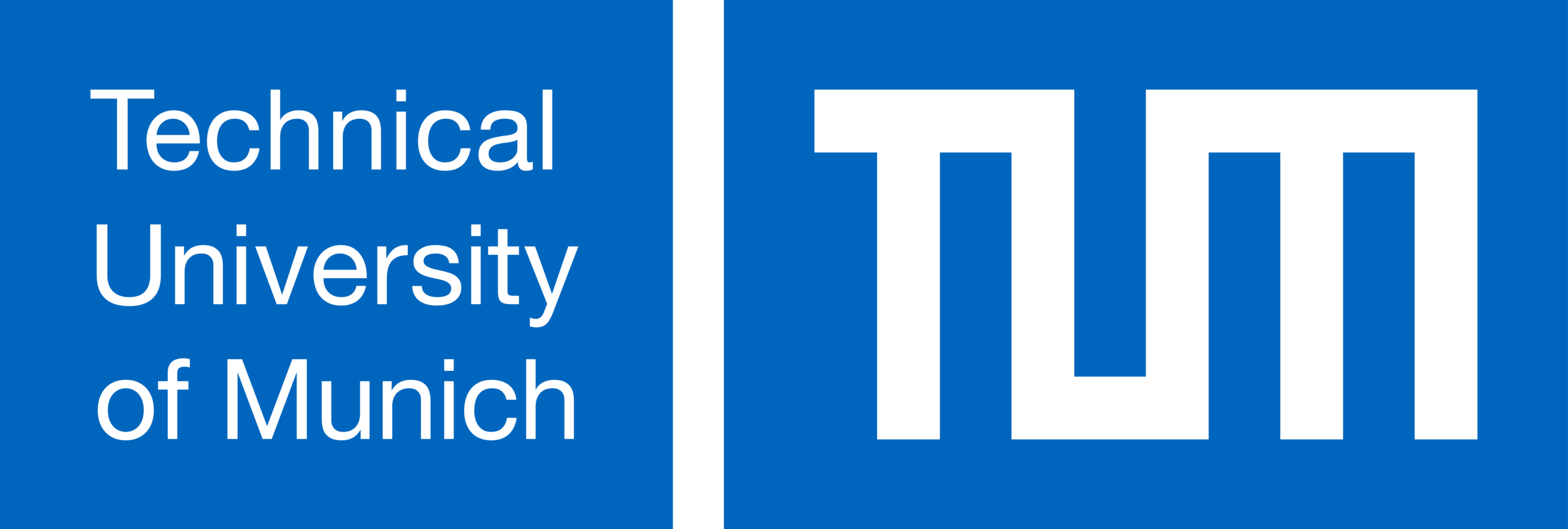
Find more about the Technical University of Munich
Artery-on-a-chip to investigate cardiovascular diseases and therapeutic targets
Investigating etiopathogenetic mechanisms underlying cardiovascular diseases (CVD) and the study of disease progression and novel therapies have been made possible through in-vivo animal models. However, translating these findings across species can be challenging due to the unique nature of the human circulatory system.
In response, the development of organ-on-a-chip (OOC) models has emerged to bridge the gap between animal and human models by mimicking key aspects of human physiology.
OOC models are micro-engineered in vitro replicas of human organs, allowing for pathophysiological studies. These models consist of microfluidic cell culture devices with continuously perfused chambers, housing living cells arranged to mimic tissue geometry. The integration of patient-derived cells and precise environmental control enhances our ability to discover novel targets and conduct drug testing.
In a recent study, artery-on-a-chip technology was applied to investigate two types of cardiovascular diseases: carotid artery disease (CAD) and abdominal aortic aneurysms (AAA).
CAD involves atherosclerosis, which narrows arterial lumens and reduces blood flow to the brain, potentially causing transient ischemic attacks or strokes. Most CAD lesions occur at the carotid bifurcation, where complex wall shear stress distributions are present. AAA, on the other hand, results from the pathological widening of the aortic lumen and can lead to rupture with high mortality rates. Most AAA cases develop in the infrarenal segment of the aorta.
Despite their differences, carotid artery disease and abdominal aortic aneurysms share common characteristics, including pathological remodeling of the vessel wall and alterations in hemodynamic shear stresses. The endothelial layer, at the interface with blood flow, plays a critical role in both diseases. Subsequent remodeling of the medial layer, populated by smooth muscle cells (SMC), leads to disease progression. CAD is marked by plaque buildup, intima layer disappearance, and increased inflammation. In aneurysms, the vessel wall becomes dysfunctional due to the loss of vascular SMC and the destruction of elastic matrix fibers.
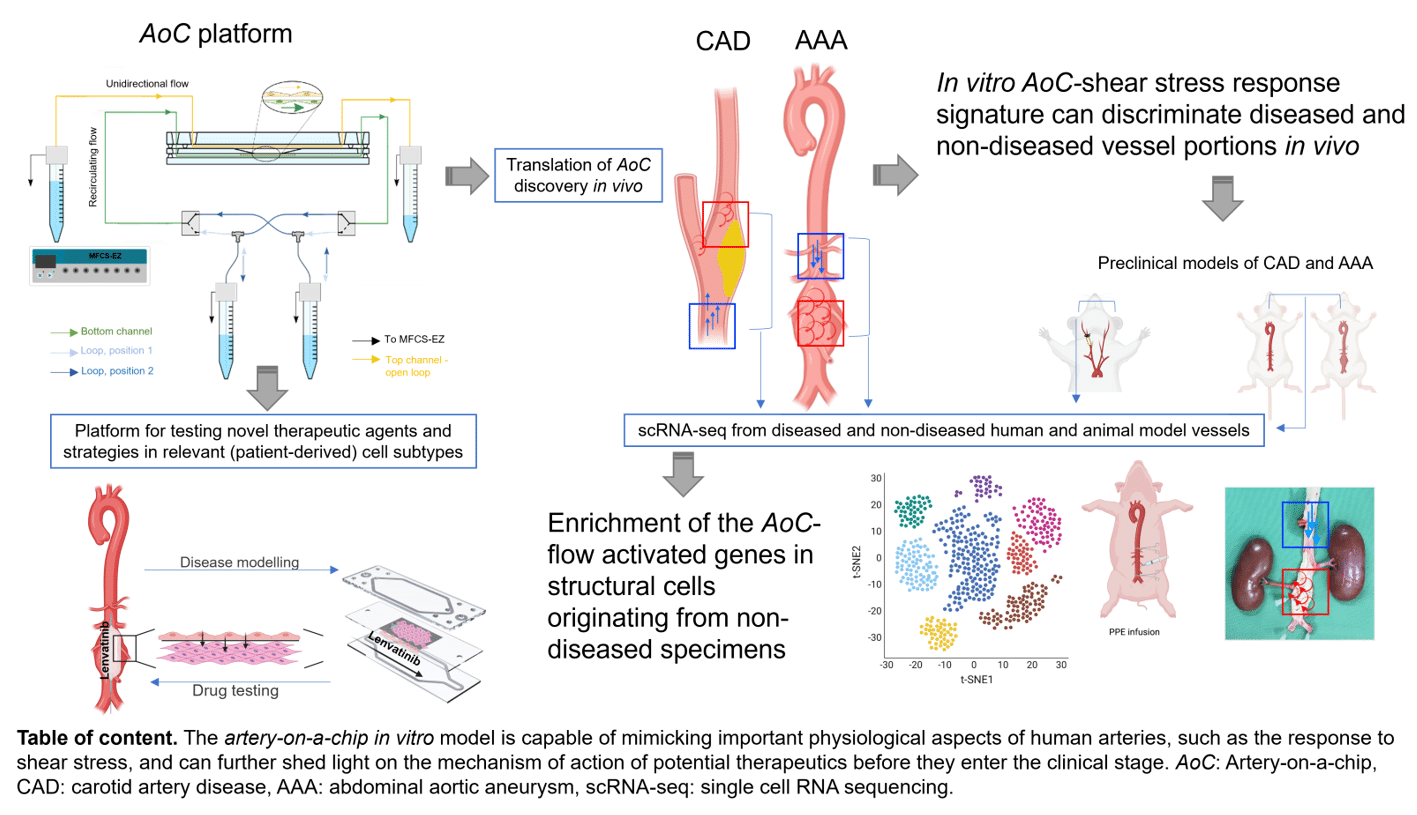
Figure 1. Summary of the study. AoC: Artery-on-a-chip. CAD: carotid artery disease, AAA: abdominal aortic aneurysm, scRNA-seq: single cell RNA sequencing.
The study introduces the artery-on-a-chip (AoC) model, which mimics the arterial wall structure and explores interactions between vascular endothelial cells (EC) subjected to shear stress and underlying SMC cultured on a layer of extracellular matrix. The AoC serves as a valuable tool for molecular target discovery in cardiovascular disease research, and the findings have the potential to translate into the context of human diseases. Additionally, the artery-on-a-chip can be used for drug testing, allowing researchers to evaluate the effects of therapeutic agents on cellular and molecular pathophysiology, potentially advancing the development of treatments for CAD and AAA.
This table of content summarizes the entire study and its major findings.
The artery-on-a-chip in vitro model is capable of mimicking important physiological aspects of human arteries, such as the response to shear stress, and can further shed light on the mechanism of action of potential therapeutics before they enter the clinical stage.
Use of the MFCS-EZ in the Artery-on-a-chip model
Experimental Design: Artery-on-a-Chip
The device employed in this study was collaboratively designed with Micronit Technologies (Enschede, The Netherlands). It comprised a membrane layer and two resealable glass slides, constituting the upper and lower chambers of the Artery-on-a-chip device. Cells utilized on the AoC were primary human aortic endothelial cells (EC) and human aortic smooth muscle (SMC), both derived from healthy donors (Cell Application, CA, USA). The assembled AoC was firmly affixed within the chip holder, a cell culture platform, with distinct control over fluid flows both above and below. Fluid regulation was managed through the connection, facilitated by a perfusion set linked to Fluigent’s microfluidic pressure-based pump (MFCS-EZ). To ensure a consistent flow rate throughout the experiment, flow sensors were strategically placed between the reservoirs and the AoC. A continuous regulation algorithm monitored the ongoing flow rates, dynamically adjusting the pressure in response to fluctuations. Installation of flow sensors between the reservoirs and the artery-on-chip facilitated precise monitoring of the flow rate. At the end of the flow experiment, (24 hours after flow exposure) the AoC was disassembled, the membrane was rinsed and processed for subsequent testing protocols.
Partial results
The robustness of this study relies on their human vascular biobank. The outcomes derived from the Artery-on-a-Chip (AoC) system were further authenticated through validation in samples obtained from vascular tissues of individuals afflicted by carotid atherosclerosis and abdominal aortic aneurysms (AAA).
The AoC: An In Vitro Model of the Arterial Wall
The artery-on-a-chip model comprises a resealable glass chip with two flow channels separated by an intermediate layer that contains a porous culture membrane. This membrane facilitates the circulation of different fluids on either side, allowing for co-culture at an interface (Figure 1.A). Primary aortic endothelial cells (EC) are grown on one side of the membrane on a collagen layer, while primary aortic smooth muscle cells (SMC) are cultured on the other side on a fibronectin layer (Figure 1.B). Immunofluorescence staining using specific antibodies validated the quality of the co-culture, confirming the attachment of EC and SMC at opposing sides of the membrane (Figure 1.C).
Once cells reach confluence, the glass chip is assembled, sealed, and connected to Fluigent’s MFCS, microfluidic pressure controller, enabling perfusion with cell-specific culture medium. The AoC is integrated into an incubator and perfused for 24 hours at a steady flow rate, maintaining a constant wall shear stress (WSS) of 10 dyne/cm² on the EC channel. The flow rate in the SMC channel, designed to mimic physiological conditions, remains minimal (10 μL/min), generating a negligible shear stress (0.0021 dyne/cm²) necessary for nutrient turnover and waste removal. Flow sensors and specific valves ensure a constant flow rate and unidirectional recirculation of EC medium between reservoirs connected to the EC channel, establishing a controlled and stable microenvironment within the AoC model (Figure 1.D).
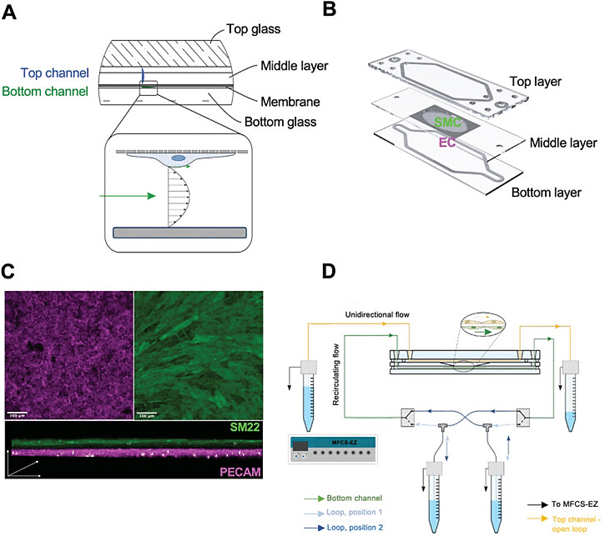
Figure 2. The Artery-on-Chip model. A) Cross-sectional design showing the layers of the AoC. B) The membrane for co-culturing endothelial cells EC and smooth muscle cells SMC at opposite sides is inserted between the top and bottom layer. C) Immunofluorescence membrane staining showing EC and SMC, labeled by the respective cell markers (PECAM for EC and SM22 for SMC). D) Connection of the AoC to the Fluigent microfluidic pressure controller (MFSC-EZ).
AoC relevance to in-vivo: change in gene expression levels
In this study, the assembled Artery-on-a-Chip (AoC) model was evaluated under shear stress conditions, maintaining a constant wall shear stress (WSS) of 10 dynes/cm², as opposed to static conditions. The findings revealed its capability to identify biologically relevant flow-inducible genes. This study verified in vitro flow-induced expression of CRYAB and INHBA in non-diseased vessels obtained from patients, confirmed at both the RNA and protein levels. INHBA, encoding the inhibin𝛽A subunit of Activin A, a multifunctional cytokine inhibiting vascular endothelial cell growth, aligned with a quiescent endothelial cell (EC) monolayer exposed to physiologically relevant shear stress.
Similarly, INHBA was among the genes expressed by endothelial cells under prolonged unidirectional pulsatile flow. The increased expression of CRYAB, observed both in the in vitro model and non-diseased human vessels, may indicate a protective role, given its function in stabilizing the cytoskeleton in response to mechanical stress. These findings support the hypothesis that a selected wall shear stress (WSS) of 10 dyne/cm² exhibits a vasoprotective effect on EC in vitro, suggesting translatability to human specimens. Immunofluorescence confirmed the presence of CRYAB and INHBA in the healthy segments of carotid arteries and aortas, co-localizing with both EC and smooth muscle cell markers.
Overall, the loss of CRYAB and INHBA expression seems to have detrimental effects on the homeostasis of SMC in the arterial wall in human disease and experimental models, while being associated with vascular and immune system dysfunction.
AoC Serves as a Drug Testing Device for Novel Therapies in Vascular Diseases
To explore the potential of the Artery-on-a-Chip model as a drug-testing device for vascular diseases, they investigated its ability to mimic in vivo conditions, particularly the intima-blood flow interface (Figure 2).
Employing the AoC, they tested the impact of Lenvatinib, a tyrosine kinase inhibitor known for limiting aortic aneurysm expansion in preclinical murine and porcine models. In their experiments, the endothelial cell (EC) layer of the AoC was exposed to circulating medium containing Lenvatinib or a control (DMSO), while smooth muscle cells (SMC) were exposed to SMC medium.
SMC, isolated from patients with aortic aneurysms, exhibited an upregulation of genes associated with a contractile phenotype when co-cultured with EC exposed to Lenvatinib. Notably, Lenvatinib treatment induced downregulation of VEGFR2, PECAM, and VCAM1 in EC, aligning with its inhibitory role in angiogenesis and anti-inflammatory effects.
These results mirror in vivo observations, emphasizing the potential of the AoC model for future clinical studies in aortic aneurysm patients.
Testimonial
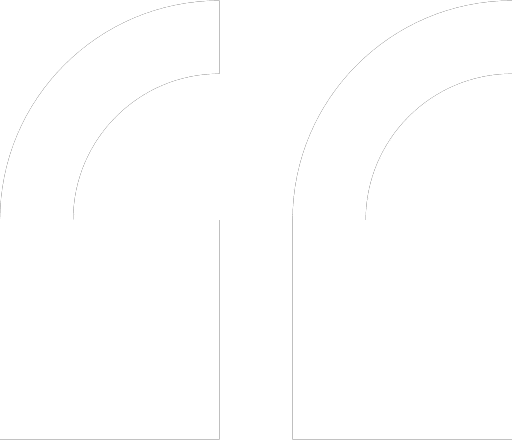
“The setup of our working model hasn’t been trivial, and we certainly wouldn’t have succeeded without the support of Fluigent. Together with the R&D engineer, Felix Rogowitz, we made specific adjustments to our flow setup which improved stability and reproducibility of our experiments. It has been a hands-on journey with the Fluigent team, and we are very thankful for the fruitful collaboration.”
Dr. Valentina Paloschi
Conclusion
The Artery-on-a-Chip model, designed to replicate the arterial vessel wall’s structural and hemodynamic aspects, serves as an innovative tool in cardiovascular research. Bridging the gap between cell culture and animal models, the AoC enhances the ability to emulate complex human vascular biology, providing insights into novel targets and validating known flow-responsive genes.
The system demonstrates translational potential by serving as a platform for testing therapeutic agents in patient-derived cell subtypes. This is particularly significant in adhering to the 3R criteria in animal research and contributes to drug target discovery and testing in cardiovascular disease research.
The AoC’s utilization of primary patient-derived cells and induced pluripotent stem cells (iPSCs) holds promise for investigating syndromic forms of cardiovascular diseases in the future, such as Marfan Syndrome or Vascular Ehlers-Danlos Syndrome.
Full article
Paloschi V, Pauli J, Winski G, Wu Z, Li Z, Botti L, Meucci S, Conti P, Rogowitz F, Glukha N, Hummel N, Busch A, Chernogubova E, Jin H, Sachs N, Eckstein HH, Dueck A, Boon RA, Bausch AR, Maegdefessel L. Utilization of an Artery-on-a-Chip to Unravel Novel Regulators and Therapeutic Targets in Vascular Diseases. Adv Healthc Mater. 2023 Oct 5:e2302907.
Related products
Expertises & Resources
-
Microfluidics case studies Creating a Microfluidic Cancer-on-Chip Platform using Fluigent’s High Throughput Cell Perfusion Pack Read more
-
Microfluidic Application Notes Pressure predictions for lab-on-chip operations using a microfluidic network solver and Fluigent PX Read more
-
Microfluidic Application Notes Long-term fluid recirculation system for Organ-on-a-Chip applications Read more
-
Microfluidics case studies CNRS/UTC: study of a liver-on-a-chip model Read more
-
Microfluidics White Papers Microfluidic white paper – A review of Organ on Chip Technology Read more
-
Expert Reviews: Basics of Microfluidics Why Control Shear Stress in Cell Biology? Read more
-
Expert Reviews: Basics of Microfluidics Mimicking in-vivo environments: biochemical and biomechanical stimulation Read more
-
Expert Reviews: Basics of Microfluidics Application of microfluidic chip technology Read more