PLGA nanoparticle synthesis using 3D microfluidic hydrodynamic focusing
Nanoparticles can be used as drug delivery systems suitable for most administration routes. A variety of natural and synthetic polymers have been explored for the preparation of nanoparticles. Poly lactide-co-glycolic acid (PLGA) has been extensively investigated because of its biocompatibility and biodegradability (2).
These have emerged as important vehicles in scientific and biomedical research thanks to their high biocompatibility and low toxicity. One of the main advantages of PLGA is the generation of lactic and glycolic acids upon hydrolysis, endogenous metabolites that can be degraded through the Krebs cycle. This results in minimal toxicity and establishes PLGA as one of the most easily metabolized and tolerated polymers by the body (1). PLGA nanoparticles can encapsulate different API’s, from small hydrophobic and hydrophilic drugs to nucleic acids and proteins.
These can be easily conjugated to molecules modifying their charge, hydrophobicity, half-life in blood circulation and even allow selective binding to certain cell types (2).
In this application note, PLGA nanoparticle synthesis with high monodispersity and in a wide range of particle sizes has been performed, using Secoya Technologies' RayDrop single emulsion chip and Fluigent's pressure-based flow controllers. The influence of the fluidic parameters on the size of the PLGA nanoparticle is also presented.
Introduction
Polymers were first introduced three decades ago as bioresorbable surgical devices. Since then, polymer-based nanoparticles have been extensively studied. Nanocarriers formulated with biocompatible and biodegradable polymers approved by the US FDA (Food and Drug Administration) and EMA (European Medicines Agency) are being studied for the controlled delivery of various therapeutic agents (1).
Among the various polymers synthesized for formulating polymeric nanoparticles, poly(lactic-co-glycolic acid) (PLGA) is the most popular. PLGA nanoparticles have several beneficial properties such as controlled and sustained release, low cytotoxicity, long-standing biomedical applications, biocompatibility with tissues and cells, prolonged residence time and targeted delivery (6). These characteristics have accelerated the PLGA nanoparticle synthesis for use as nano-drug delivery systems (nanoDDS) in a wide variety of diseases, including cardiovascular, neurodegenerative and inflammatory and immune system diseases, infection, cancer, regenerative medicine and the fields of theragnostic and vaccines (4).
When PLGA is used as an active pharmaceutical ingredient carrier, it’s important to produce highly monodispersed particles for drug release reproducibility. PLGA nanoparticle synthesis with different characteristics (size, size distribution, morphology, zeta potential) is also possible by controlling the parameters specific to the synthesis method employed (3).
Current methods of particle synthesis rely largely on batch stirred homogenizers (single emulsion, double emulsion, etc.). However, they generally tend to have low reproducibility and are not well controlled. Some have low encapsulation efficiency and low drug loading. As narrow distributions, small particle size, and controllable synthesis are required in the field of smart drug delivery, these do not provide a highly effective solution for the pharmaceutical industry (4).
Microfluidic methods and, especially the 3D hydrodynamic flow-mediated nanoparticle production strategy of the RayDropTM make it possible to obtain a continuous PLGA nanoparticle synthesis with high monodispersity, high reproducibility and a wide range of nanoparticle size.
PLGA nanoparticle synthesis: Materials and methods
PLGA nanoparticle production has been performed with Fluigent’s Nanoparticle Production Station, a robust and complete system for precise and long-term production of nanoparticles with flexible particle size range.
PLGA Nanoparticle production setup
Reagents
Continuous phase: deionized water and 1 % Polyvinyl alcohol Mw 9000 – 10000 80% hydrolyzed (Sigma Aldrich).
Inner phase: technical acetone, and PLGA Resomer 756 1 % (Sigma Aldrich).
Inner phase to initiate and clean : technical acetone.
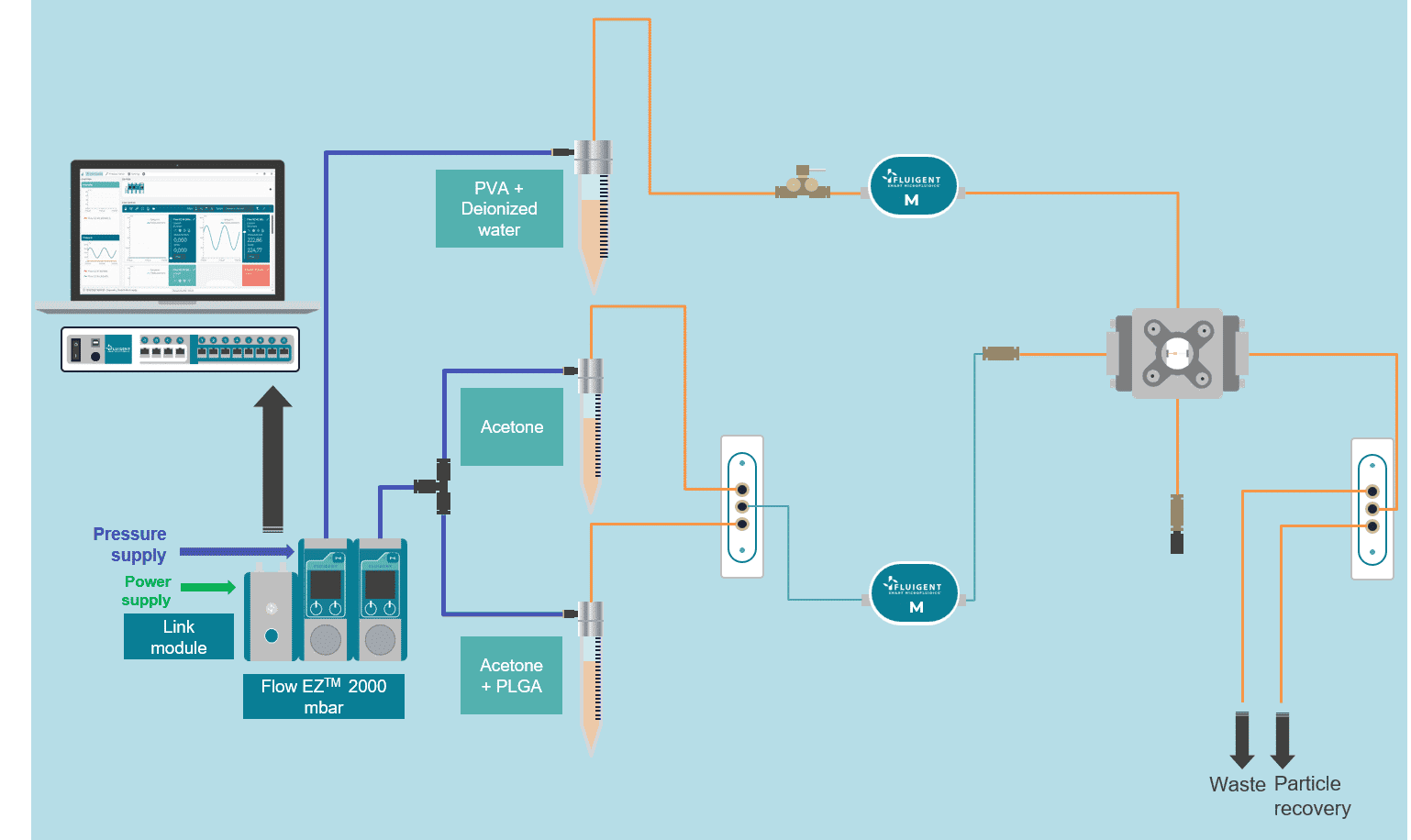
Figure 1: Scheme of the fluidic setup
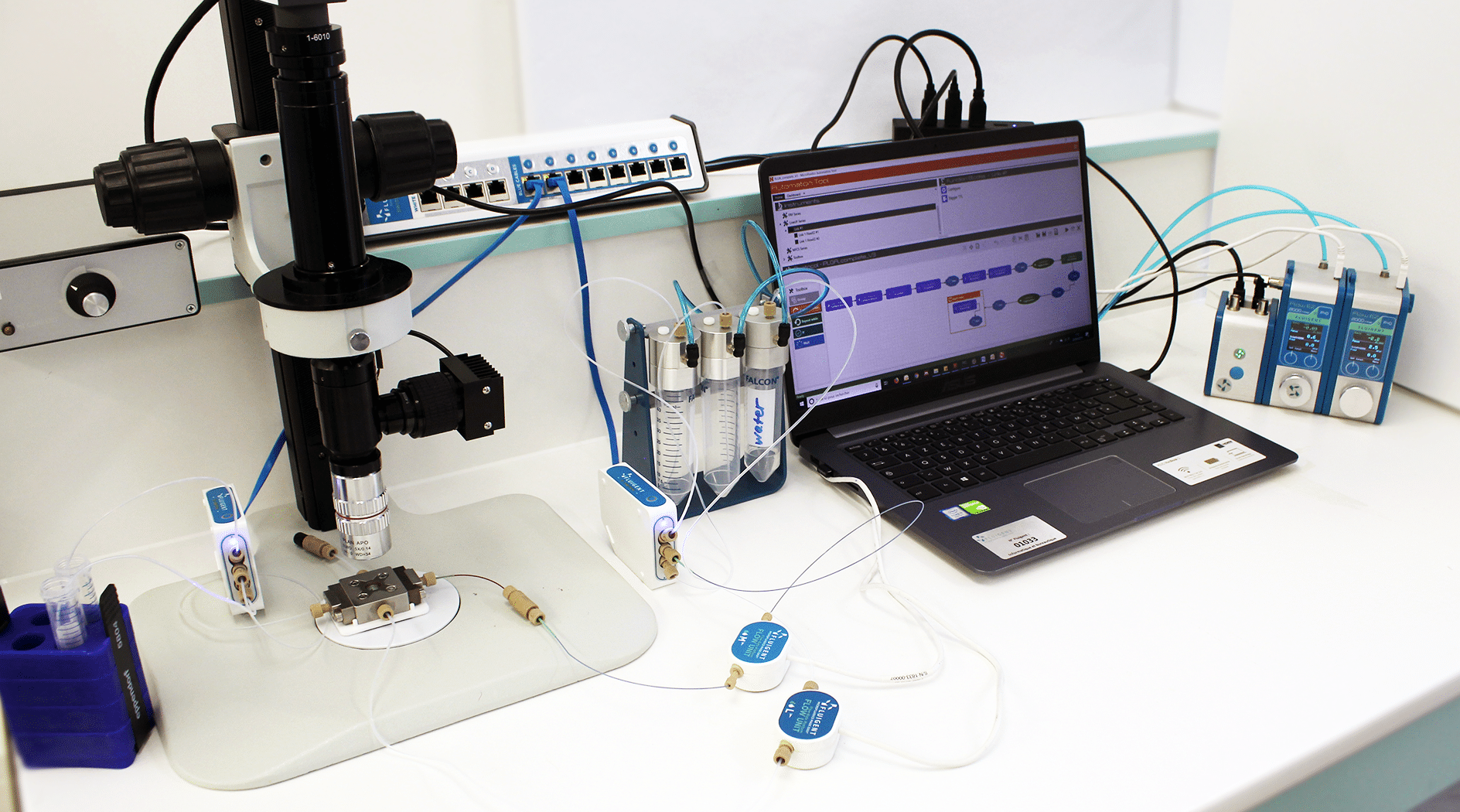
Figure 2: Picture of the Fluigent equipment
PLGA nanoparticle synthesis
In the microfluidic solvent diffusion method, nanoparticles are synthesized in a microchannel after mixing between PLGA-acetone solution and water, following a three-dimensional hydrodynamic flow focusing (3D MHF) strategy.
In this approach, flow focusing squeezes the PLGA in acetone stream between water streams fully surrounding the PLGA phase and resulting in rapid solvent exchange via diffusion and PLGA nanoparticles precipitation (9). Particle formation takes place spontaneously at the nucleation spots that are distributed through the mixture Figure 1 (10).
The reagents and precipitating NPs are isolated from the channel walls, minimizing aggregation and/or clogging. In addition, by constraining the sample stream in the center of the microchannel- where flow velocity reaches the maximum with less variation- the 3D focused sample stream is expected to have a uniform width and thus improve the uniformity of the solvent/non-solvent ratio. This allows a robust and predictable nanoparticle synthesis, and facilitates the production of highly uniform nanoscale PLGA nanoparticles (12-14).
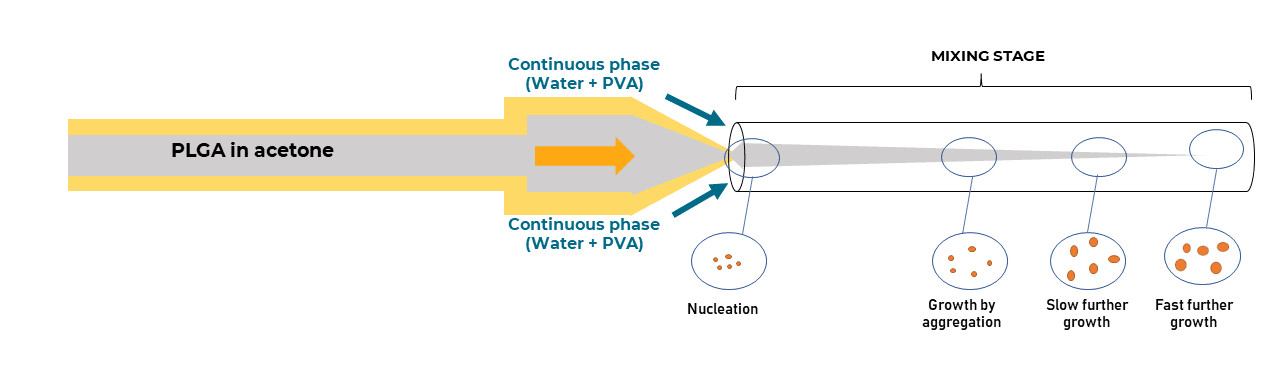
PLGA nanoparticle synthesis: Partial results
Fluigent has generated PLGA nanoparticles of different sizes by varying parameters related to our microfluidic system, thus establishing a relation between the diameter of the nanoparticle, the stream diameter, the flow rate ratio and the total flow rate.
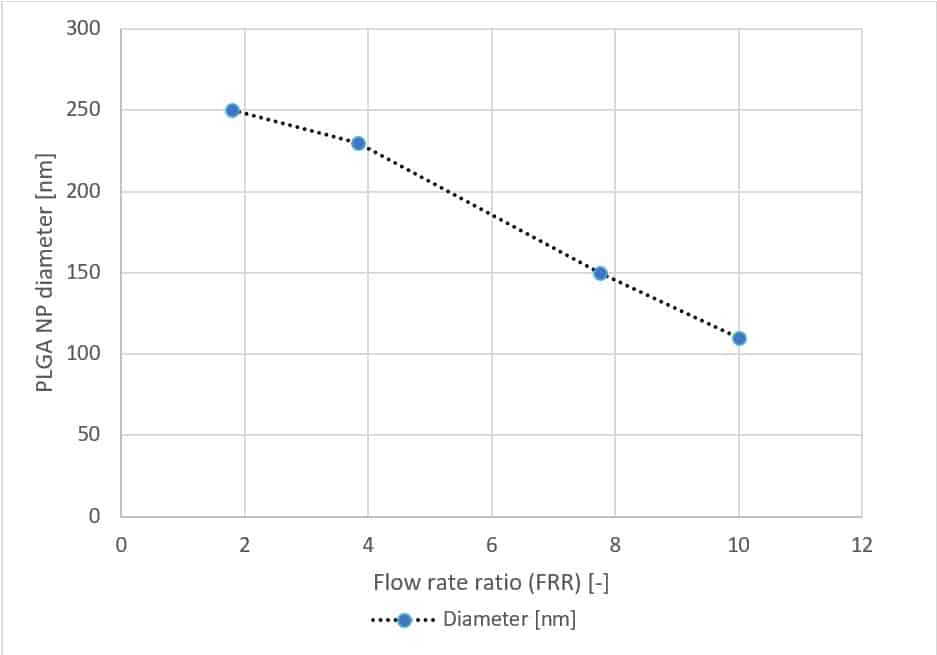
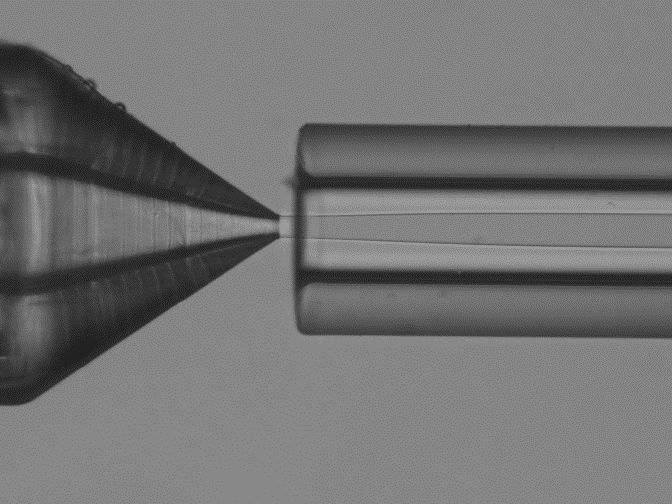
Conclusion
PLGA nanoparticles as biocompatible nanocarriers represent one of the most innovative, non-invasive approaches for drug delivery applications. However, their targeting functions are largely affected by size. In the case of tumor targeting and drug delivery, currently the commonly recognized size range for PLGA nanoparticles is 100-300 nm, as it allows for the correct targeting of nanoparticles to the desired tissue (18).
PLGA nanoparticle size may be controlled by tuning the synthesis method and parameters of operation.
In this application note, we have demonstrated the PLGA nanoparticle synthesis using a microfluidic system (3D microfluidic hydrodynamic flow) consisting of pressure-based flow controllers and the RayDrop™ microfluidic device with standard configuration.
PLGA nanoparticles ranging from 110 to 250 nm were generated. This size range is optimal for various biological applications, such as tumor targeting, as it falls within the compatible size range. The Polydispersity Index (PDI) ranges from 0.05 to 0.1. Sizes can be adjusted by controlling the device flow input parameters, particularly the flow rate ratio (FRR). In this way, the ability to synthesize PLGA nanoparticles in a more controllable and reproducible way creates possibilities for custom tuning surface properties.
A full-featured, cost-effective and readily available platform for the on-demand production of monodisperse PLGA nanoparticles is now available. This allows for control of nanoparticle size and frequency by adjusting flow parameters.
Resources & Expertises
References
- Astete, C., & Sabliov, C. (2006). Synthesis and characterization of PLGA nanoparticles. Journal Of Biomaterials Science, Polymer Edition, 17(3), 247-289. doi: 10.1163/156856206775997322
- Danhier, F., Ansorena, E., Silva, J., Coco, R., Le Breton, A., & Préat, V. (2012). PLGA-based nanoparticles: An overview of biomedical applications. Journal Of Controlled Release, 161(2), 505-522. doi: 10.1016/j.jconrel.2012.01.043
- Acharya, S., & Sahoo, S. (2011). PLGA nanoparticles containing various anticancer agents and tumour delivery by EPR effect. Advanced Drug Delivery Reviews, 63(3), 170-183. doi: 10.1016/j.addr.2010.10.008
- Senapati, S., Mahanta, A., Kumar, S., & Maiti, P. (2018). Controlled drug delivery vehicles for cancer treatment and their performance. Signal Transduction And Targeted Therapy, 3(1). doi: 10.1038/s41392-017-0004-3
- Sahoo, S., Panyam, J., Prabha, S., & Labhasetwar, V. (2002). Residual polyvinyl alcohol associated with poly (d,l-lactide-co-glycolide) nanoparticles affects their physical properties and cellular uptake. Journal Of Controlled Release, 82(1), 105-114. doi: 10.1016/s0168-3659(02)00127-x
- Rezvantalab, S., Drude, N., Moraveji, M., Güvener, N., Koons, E., & Shi, Y. et al. (2018). PLGA-Based Nanoparticles in Cancer Treatment. Frontiers In Pharmacology, 9. doi: 10.3389/fphar.2018.01260
- Tewes, F., Munnier, E., Antoon, B., Ngaboni Okassa, L., Cohen-Jonathan, S., & Marchais, H. et al. (2007). Comparative study of doxorubicin-loaded poly(lactide-co-glycolide) nanoparticles prepared by single and double emulsion methods. European Journal Of Pharmaceutics And Biopharmaceutics, 66(3), 488-492. doi: 10.1016/j.ejpb.2007.02.016
- Niwa, T., Takeuchi, H., Hino, T., Kunou, N., & Kawashima, Y. (1993). Preparations of biodegradable nanospheres of water-soluble and insoluble drugs with D,L-lactide/glycolide copolymer by a novel spontaneous emulsification solvent diffusion method, and the drug release behavior. Journal Of Controlled Release, 25(1-2), 89-98. doi: 10.1016/0168-3659(93)90097-o
- Surdo, S., Geven, M., Donno, R., Diaspro, A., Tirelli, N., & Duocastella, M. (2018). Cavitation-Assisted Micromixing for Polymeric Nanoparticle Generation. EUROSENSORS 2018. doi: 10.3390/proceedings2130942
- Karnik, R., Gu, F., Basto, P., Cannizzaro, C., Dean, L., & Kyei-Manu, W. et al. (2008). Microfluidic Platform for Controlled Synthesis of Polymeric Nanoparticles. Nano Letters, 8(9), 2906-2912. doi: 10.1021/nl801736q
- Rezvantalab, S., & Keshavarz Moraveji, M. (2019). Microfluidic assisted synthesis of PLGA drug delivery systems. RSC Advances, 9(4), 2055-2072. doi: 10.1039/c8ra08972h
- Rhee, M., Valencia, P.M., Rodriguez, M.I., Langer, R., Farokhzad, O.C. and Karnik, R. (2011), Synthesis of Size-Tunable Polymeric Nanoparticles Enabled by 3D Hydrodynamic Flow Focusing in Single-Layer Microchannels. Adv. Mater., 23: H79-H83. https://doi.org/10.1002/adma.201004333
- Lim, J., Bertrand, N., Valencia, P., Rhee, M., Langer, R., & Jon, S. et al. (2014). Parallel microfluidic synthesis of size-tunable polymeric nanoparticles using 3D flow focusing towards in vivo study. Nanomedicine: Nanotechnology, Biology And Medicine, 10(2), 401-409. doi: 10.1016/j.nano.2013.08.003
- Génot, V., Desportes, S., Croushore, C., Lefèvre, J., Pansu, R., Delaire, J., & von Rohr, P. (2010). Synthesis of organic nanoparticles in a 3D flow focusing microreactor. Chemical Engineering Journal, 161(1-2), 234-239. doi: 10.1016/j.cej.2010.04.029