Microfluidic technology for engineered nanoparticles in nanomedicine
Engineered Nanoparticles (NPs) are becoming an essential tool to enhance drug delivery systems for both biomedical and pharmaceutical applications. Controlling the delivery of molecules of interest increases their concentration in a desired location and leads to improved drug efficacy while limiting adverse effects. NPs, including lipid-based and polymeric NPs, have been used over the years as nanocarriers of various bioactive molecules.
In this paper highlight, the authors review the conventional formulations and methods used to manufacture polymeric and lipid-based NPs, including microfluidic-based engineered nanoparticle production, and consider their respective advantages and limitations.
The Lamprou Lab (Queen’s University Belfast)
The Lamprou lab, affiliated with Queen’s University Belfast, specializes in three main areas: nanoparticles for imaging and therapy, lab-on-a-chip technology, and therapeutic implants. Their interdisciplinary approach has driven innovation in healthcare since 2012, developing emerging technologies and novel drug delivery devices. The lab is led by Professor Dimitrios Lamprou, a leading expert in pharmaceutical technologies known for his significant contributions to 3D printing, microfluidics, and nanofibers, with over 150 peer-reviewed publications.
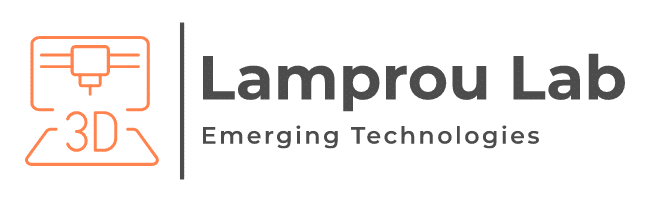
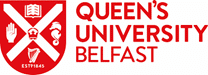
What are engineered nanoparticles?
Nanotechnology gained prominence after Richard P. Feynman’s 1959 famous lecture, “There’s plenty of room at the bottom”.1 However, the use of nanoparticles can be traced back to ancient times, as seen in the Romans’ incorporation of nanoparticles into glass manufacturing in the fourth century AD. The Lycurgus cup, an artifact from this period, notably displayed distinctive color changes under various lighting conditions due to the integration of nano-glass particles.2
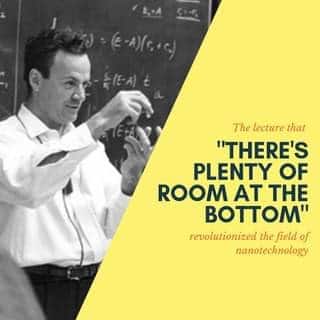
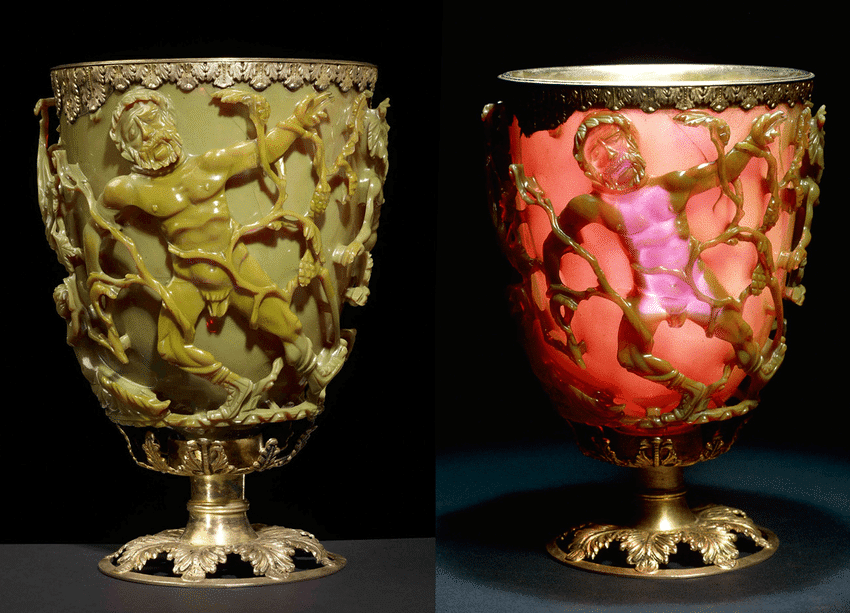
In modern times, nanotechnology has developed into a comprehensive scientific discipline with diverse applications spanning multiple industries. From water purification and information technologies to drug development, environmental solutions, and the creation of robust yet lightweight materials, nanotechnology has emerged as a pivotal player.3,4
The fundamental units of nanotechnology are nanoparticles, defined as small particles ranging from 10 nm to 1000 nm in size. Engineered nanoparticles, specifically designed with dimensions under 100 nm, play a crucial role in manipulating materials at the molecular and atomic levels, demonstrating significant chemical, structural, electrical, biological and mechanical characteristics. They are classified into categories including ceramic, carbon-based, semiconductor, metal, lipid-based and polymeric nanoparticles.
What makes engineered nanoparticles promising in nanomedicine?
Engineered nanoparticles hold promise for various nanotechnology applications in medicine, including in-vivo and in-vitro diagnosis, drug delivery, and production of biocompatible materials. They are characterized by their high mass-to-surface area ratio, ability to adsorb and carry compounds, and quantum properties.5,6
These characteristics can benefit the drug delivery field, as its primary objective is to enhance the specificity of drug targeting, improve safety and biocompatibility, and reduce toxicity while maintaining therapeutic effects. Nanoparticles with dimensions less than 100 nm are considered excellent drug carriers due to their unique biological and physiological properties, allowing them to cross tissue and cell barriers effectively.7
Engineered nanoparticles also exhibit a higher likelihood of cellular uptake due to their larger surface area, enabling increased protein loading. The interaction of NPs with biological substances results in the formation of a “protein corona,” enhancing nanocarriers’ uptake by the reticuloendothelial system. This protein corona can serve as a functional carrier for targeted drug delivery, improving poorly soluble drug uptake, directing drugs to specific locations, and enhancing drug bioavailability.8
Overall, engineered nanoparticle applications in nanomedicine have been progressing recently, particularly in controlled drug delivery, nucleic acid-based treatment, cancer cell targeting, angiogenesis inhibition, and inflammation control.
How are engineered nanoparticles traditionally produced
The success of nanoparticles as drug carriers and in nanomedicine applications depends on a number of crucial factors, including NP fabrication strategies, physical properties, drug loading efficiencies, drug release potential, and especially the carrier’s toxicity.
Lipid-based nanoparticles exhibit low toxicity in in-vivo experiments. They can carry both hydrophilic and hydrophobic molecules, leading to prolonged half-life and controlled drug release. Here, we focus on two main classes of lipid-based nanoparticles: liposomes and solid lipid nanoparticles.
Liposomes
A liposome is a microsphere lipid constructed from one or multiple phospholipid bilayers, closely mirroring the structure of cell membranes. The liposome preparation process consists of three main stages: preparing aqueous and lipid phases, primary processing with lipids, and optional secondary processing steps. Most methods involve dissolving phospholipids in an organic solvent, with subsequent removal of the solvent through evaporation—a critical step in liposome formation. Two straightforward methods of liposome synthesis are film hydration and solvent injection.
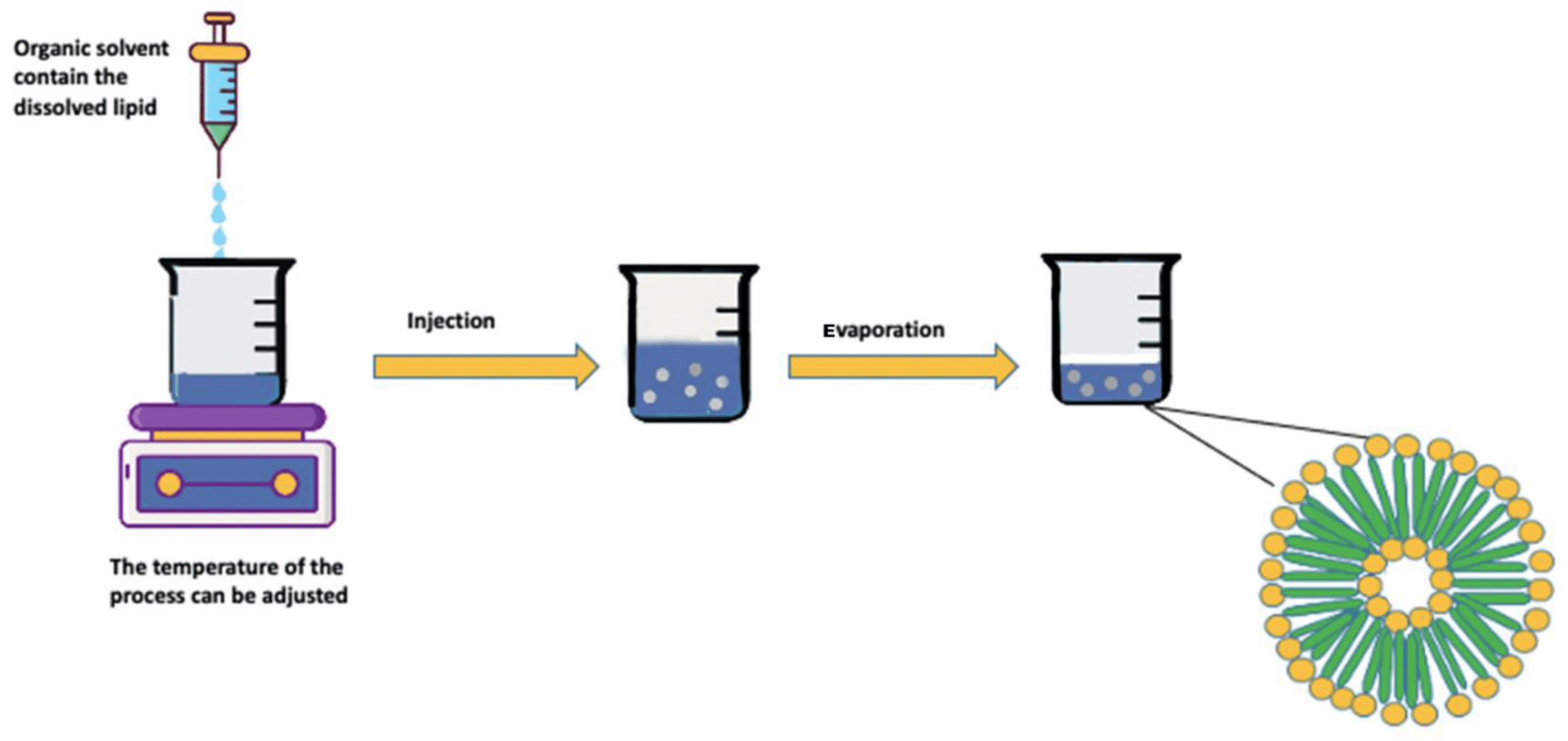
Liposomes generated through film hydration tend to be polydisperse. Parameters like the duration of rotary evaporation, mixing speed, and temperature after hydration affect liposome quality, emphasizing the need for careful monitoring.10,11
The major factors to consider for use of the solvent injection method are the temperature during injection and the injection rate. These factors will affect the size, shape, and polydispersity of the liposomes produced. The solvent injection method involves certain challenges, with continuous exposure of therapeutic substances to high temperatures and organic solvents affecting liposomal product stability and safety.
This results in high polydispersity and a non-homogeneous formulation.12
Solid Lipid Nanoparticles
Solid lipid nanoparticles (SLN) have emerged as a promising alternative to other lipid formulations like liposomes or polymeric nanoparticles. These spherical colloidal particles, ranging from 10 to 1000 nm in size, offer controlled drug release due to limited drug mobility in the solid lipid. SLNs provide advantages such as targeted drug delivery, controlled release, increased stability, scalability in production, and avoidance of organic solvents. Comprising a solid lipid core in an aqueous medium with a surfactant, SLNs use different lipids (steroids, fatty acids, triglycerides, etc.) and require stabilizing agents like surfactants or emulsifiers. The drug insertion process depends on drug hydrophobicity, solid lipid category, and polymeric alterations in the lipid. Production techniques include high-pressure homogenization, solvent evaporation, ultrasonication, hot homogenization, microemulsion, and others.13,14
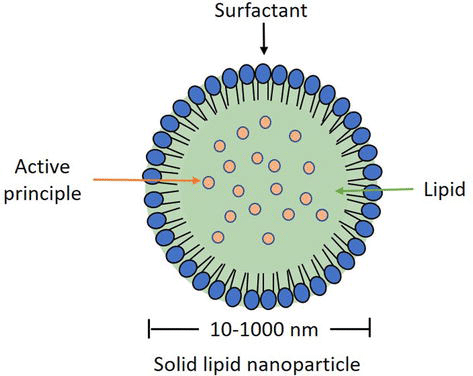
Table: comparison of the different manufacturing methods used for polymeric NPs and lipid-based NPs
Nanoparticles Type | Manufacturing Method | Advantages | Disadvantages |
---|---|---|---|
Lipid formulation | Film hydration | – Established method – Understood method | – High consuming of the organic solvents – High PDI – Lack of reproducibility – Need for additional downsizing step – Difficulties in scaling-up |
Lipid formulation | Solvent injection | – Simple and fast – Scaling-up possibility | – Exposing to organic solvent – High PDI – Stability problems |
Lipid formulation | Extrusion | – Uniform and homogenous formulation | – Possible clogging of the membrane pores. – Difficulties in scaling-up |
Lipid formulation | High pressure homogenization | – Scaling-up possibility – Uniform formulation | – High energy consumption – Multiple steps – Bulky system |
Lipid formulation | Microemulsion | – Small particle size – Homogenous formulation | – Difficulty in removing the excess water – Use high concentration of surfactants |
Nanoparticles Type | Manufacturing Method | Advantages | Disadvantages |
---|---|---|---|
Polymeric | Emulsification-salting out | – Avoids surfactants and chlorinated solvents | – Need for purification steps – Encapsulate lipophilic drugs only |
Polymeric | Emulsification solvent diffusion | – Scaling-up possibility – Batch-to-batch reproducibility | – The possible diffusion of the hydrophilic drug into the aqueous phase – The need to eliminate high volume of aqueous phase from the colloidal dispersion |
Polymeric | Emulsification- evaporation | – Simple and versatile | – Risk of nanodroplets coalescence during the evaporation process – Time consuming |
Polymeric | Dialysis | – Effective and simple method – Produce polymeric nanoparticles with narrow distribution | – Time consuming – Use of high amount of dialyzing medium, which stimulate the premature release of NPs content |
Polymeric | Nonparticipation | – Simple and established method – Use low concentrations of surfactant | – Restricted for lipophilic drugs – Low polymer concentration obtained |
How does microfluidics enhance the properties of engineered nanoparticles
The principal innovation of microfluidics is the ability to transfer the traditional bulk technique to microscale fluidic chips. Solvents can be mixed within microchannels by a pumping system with continuous laminar flow. This type of flow offers high mixing quality and enhances the performance of microscale devices. The ability to adjust the flow rate ratio (FRR) and total flow rate (TFR) allows for continuous production of monodisperse and homogenous engineered nanoparticles.
Fluigent’s pressure-driven controllers and flow sensors enable this high stability and fast response in fluid flow, which is highly challenging to achieve with traditional pumping methods like peristaltic or syringe pumps. Overall, this method offers high reproducibility and low batch-to-batch variations. In addition, the method’s versatility makes the encapsulation process faster while keeping encapsulation efficiency high.
Microfluidics offers a fast, simple, single-step technique for liposome manufacturing, but challenges for large-scale production currently hinder its wide-scale implementation due to high costs.15,16
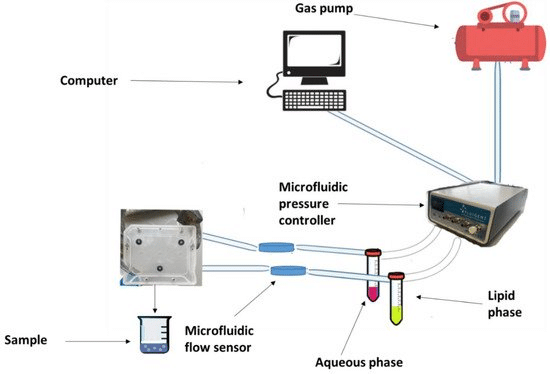
Most microfluidics-based solid lipid nanoparticle manufacturing follows a similar procedure involving dissolving lipids and drugs in an organic solvent, which is then introduced into the microfluidic device alongside an aqueous phase with a surfactant. Control over lipid-to-drug concentration, flow rate, and velocity influences the final nanocarrier characteristics.
Solid lipid nanoparticles produced by microfluidic processes have smaller particle sizes, better homogeneity, and higher encapsulation efficiency compared to bulk methods. In addition, cytotoxic studies demonstrate potent anti-proliferative effects of microfluidic SLNs in cancer cell lines.
However, research on microfluidic-produced SLNs is limited, and challenges exist, particularly with regard to the material used for microfluidic chips, with PDMS-based chips being sensitive to organic solutions.17
Conclusion
In this paper highlight, we presented the promise and challenges of microfluidics technology for the design and formulation of nanomedicines. While traditional methods face limitations in producing small engineered nanoparticles with desirable characteristics, microfluidic systems offer a one-step, controllable process with improved outcomes.
Comparative studies have shown that nanocarriers produced by microfluidics exhibit superior properties. Future advancements in microfluidic systems, combined with complementary tools like process analytical technology and molecular imaging technologies, are expected to optimize NP production and expand their medical applications.
Microfluidics holds promise for shaping the future of engineered nanoparticle research and development.
Related Products
Expertises & Resources
References
(1) Shirai, Y.; Osgood, A.J.; Zhao, Y.; Yao, Y.; Saudan, L.; Yang, H.; Yu-Hung, C.; Alemany, L.B.; Sasaki, T.; Morin, J.-F. Surface-rolling molecules. J. Am. Chem. Soc. 2006, 128, 4854–4864.
(2) Bayda, S.; Adeel, M.; Tuccinardi, T.; Cordani, M.; Rizzolio, F. The History of Nanoscience and Nanotechnology: From Chemical-Physical Applications to Nanomedicine. Molecules 2019, 25, 112. Schoenmaker L, et al. mRNA-lipid nanoparticle COVID-19 vaccines: structure and stability. Int Pharm. 2021;601: 120586 .
(3) Grobert, N.; Hutton, D. Nanoscience and nanotechnologies: Opportunities and uncertainties. Lond. R. Soc. R. Acad. Eng. Rep.2004, 46, 618.
(4) Thiruvengadam, M.; Rajakumar, G.; Chung, I.M. Nanotechnology: Current uses and future applications in the food industry.3 Biotech 2018, 8, 74.
(5) Duncan, R. The dawning era of polymer therapeutics. Nat. Rev. Drug Discov. 2003, 2, 347–360.
(6) De Jong, W.H.; Borm, P.J. Drug delivery and nanoparticles:applications and hazards. Int. J. Nanomed. 2008, 3, 133–149.
(7) LaVan, D.A.; McGuire, T.; Langer, R. Small-scale systems for in vivo drug delivery. Nat. Biotechnol. 2003, 21, 1184–1191.
(8) Nel, A.E.; Mädler, L.; Velegol, D.; Xia, T.; Hoek, E.M.; Somasundaran, P.; Klaessig, F.; Castranova, V.; Thompson, M. Understanding biophysicochemical interactions at the nano-bio interface. Nat. Mater. 2009, 8, 543–557.
(9) Mitchell, M.J., Billingsley, M.M., Haley, R.M. et al. Engineering precision nanoparticles for drug delivery. Nat Rev Drug Discov 20, 101–124 (2021).
(10) Jaradat, E.; Weaver, E.; Meziane, A.; Lamprou, D. A. Microfluidics Technology for the Design and Formulation of Nanomedicines. Nanomaterials 2021, 11 (12), 3440.
(11) Alam, S.; Mattern-Schain, S.; Best, M. Targeting and triggered release using lipid-based supramolecular assemblies as medicinal nanocarriers. In Comprehensive Supramolecular Chemistry II; Elsevier: Oxford, UK, 2017; pp. 329–364.
(12) Maherani, B.; Arab-Tehrany, E.; Mozafari, M.R.; Gaiani, C.; Linder, M. Liposomes: A review of manufacturing techniques and targeting strategies. Curr. Nanosci. 2011, 7, 436–452.
(13) Mehnert, W.; Mäder, K. Solid lipid nanoparticles: Production, characterization and applications. Adv. Drug Deliv. Rev. 2001, 47,165–196.
(14) Uner, M.; Yener, G. Importance of solid lipid nanoparticles (SLN) in various administration routes and future perspectives. Int. J.Nanomed. 2007, 2, 289–300.
(15) Weaver, E.; Uddin, S.; Cole, D.K.; Hooker, A.; Lamprou, D.A. The Present and Future Role of Microfluidics for Protein and Peptide-Based Therapeutics and Diagnostics. Appl. Sci. 2021, 11, 4109.
(16) Guimarães Sá Correia, M.; Briuglia, M.L.; Niosi, F.; Lamprou, D.A. Microfluidic manufacturing of phospholipid nanoparticles: Stability, encapsulation efficacy, and drug release. Int. J. Pharm. 2017, 516, 91–99.
(17) Arduino, I.; Liu, Z.; Rahikkala, A.; Figueiredo, P.; Correia, A.; Cutrignelli, A.; Denora, N.; Santos, H.A. Preparation of cetylpalmitate-based PEGylated solid lipid nanoparticles by microfluidic technique. Acta Biomater. 2021, 121, 566–578.