Generation of microcapsules with a UV-crosslinked polymer
Using the double emulsion RayDrop developed and manufactured by Secoya, controlled double emulsification is achieved by dripping or jetting the core fluid into an immiscible shell fluid, which is then encapsulated by the third fluid. In this note, capsules are formed by consolidating shell phase of the resulting double emulsions by uv-crosslinking of monomers and photoinitiator used as shell phase.
Overview
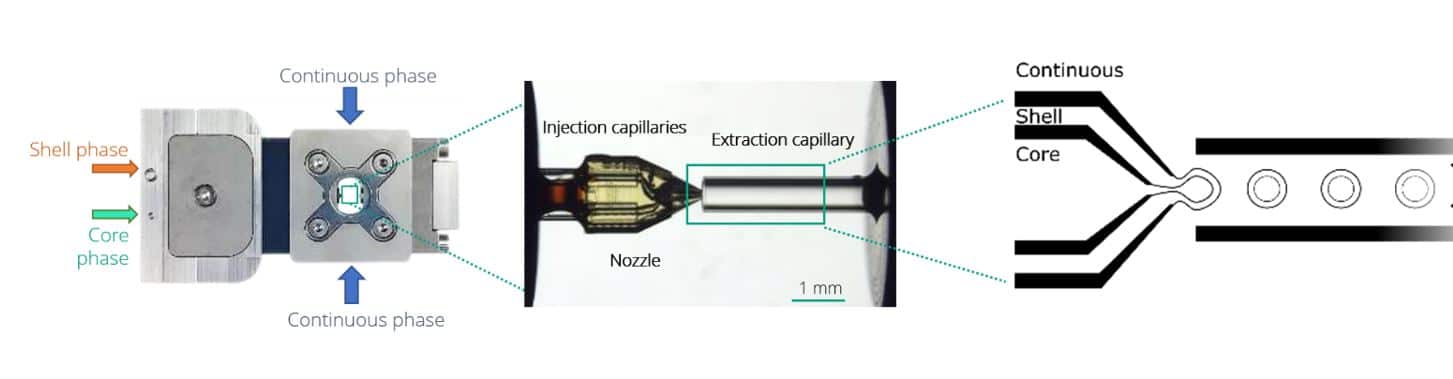
Over the past few decades, core-shell microcapsules have played an important role in the delivery and release of materials in the pharmaceutical, cosmetic, and food industries. Such applications often require a high degree of control over chemical release, a quality directly related the capsule material as well as the homogeneity of the capsule size and shell thickness.
Capsules can be produced using a variety of methods, including polymerization, spray drying, solvent evaporation, and self-assembly. These methods typically lead to high polydispersity core–shell particles [1] [2]. There is also limited control over morphology, poor reproducibility and compatibity with high encapsulation efficiencies.
Double emulsions generated using microfluidic devices has been shown to overcome these difficulties by enabling fine control of the capsule size and shell thickness, while producing highly monodispersed, perfectly spherical microparticles. Among these devices, the Raydrop is the first to allow an easy to set up and robust production of double emulsion. It doesn’t rely on double coating treatment of PDMS/glass in planar chip [3][4] or the alignment of two round capillaries in a third square tubing, as in lab-made glass capillary microfluidic device [5] [6].
As described on Figure 1, the Raydrop relies on the use of a 3D-printed injection nozzle carrying two fluids, the core and the shell phases. This is positioned in front of an extraction capillary in a cavity filled with the third (continuous) phase.
Figure 1: Double emulsion production principle
Experimental procedure
Using the double emulsion Raydrop, controlled double emulsification is achieved by dripping or jetting the core fluid into an immiscible shell fluid, which is then encapsulated by the third fluid. In this note, capsules are formed by consolidating shell phase of the resulting double emulsions by uv-crosslinking of monomers and photoinitiator used as shell phase.
Core and continuous phases are aqueous phases, each being non-miscible with the shell. Fine control of the fluid flows leads to defined capsule and shell dimensions. In-situ polymerization is achieved, meaning that the droplets are exposed to uv-light while still being moving forward in the output tubing connected to the Raydrop. Hard shell microcapsules are directly collected in the collection vial. The in-situ process avoids coalescence and deformation of the droplets that can arise in an ex-situ process where the droplets are polymerized after collection.
Figure 2: Scheme of the microcapsule production process
As the core phase, deionized water is used and for the continuous phase, 1% wt aqueous solution of Tween 20. For the shell phase, a solution of commercial Allnex methacrylate-based resin with 0.1% wt of photoinitiator Diphenyl (2,4,6-trimethylbenzoyl)phosphine oxide (TPO) from Sigma-Aldrich and 20% ethyl acetate is used.
As shown on the scheme in Figure 3, reservoirs of resin solution and pure ethyl acetate are each connected to a valve. Ethyl acetate is first used as shell phase to initiate the double emulsion. When the system is stabilized, the valve is switched to the resin polymer. At the end of the process, ethyl acetate is flushed again to rinse the nozzle and tubing. The flushing of reactive or non-miscible species after an experiment is key to maintaining good operation of the system day after day.
Double emulsions are formed by pumping the three fluids through the Raydrop using a pressure controller (Fluigent, France). The flowrates are monitored using flowmeters (Fluigent, France). When polymer double emulsions are formed, the UV-light placed above the output tubing is tuned on and the solid shell microcapsule are collected in a vial. When the experiment is over, the light source is turned off and the pure ethyl acetate is pumped as shell phase to rinse the system.
Figure 3: Setup description for microcapsule production
Results
The outer diameter of the emulsions and thus the capsule size can be selected within a broad range by changing the size of the collector capillary and the nozzle tip dimensions. This is easily achieved by a change of the two inserts.
For a configuration with the nozzle and output capillaries (respectively 90µm and 450µm ), as presented in this note, adjusting the flowrates of the fluids allows for fine control of the capsule dimensions (see Figure 4). With this setup droplet from 200µm to 300µm can be easily produced.
The shell thickness of microcapsules can also be varied by changing the ratio of flowrates of the shell and core phases, as shown in Figure 4. Here shell thickness varies from 10µm to 50µm.
Figure 4: Microcapsule of 250µm with shell thickness adjustment between 10µm to 50µm
Conclusion
In this short note we have demonstrated a solution for production of microcapsules in a controlled, reproducible manner. From droplet production to microcapsule polymerization every step is presented to allow a good reproduction of the experience. This system allows for production of highly monodisperse microcapsule (CV<2%) without interruption as compared to other methods used for microcapsule synthesis.
References
[1] Chen, P. W., Erb, R. M., & Studart, A. R. (2012). Designer polymer-based microcapsules made using microfluidics. Langmuir, 28(1), 144–152. https://doi.org/10.1021/la203088u
[2] Galogahi, F. M., Zhu, Y., An, H., & Nguyen, N. T. (2020). Core-shell microparticles: Generation approaches and applications. Journal of Science: Advanced Materials and Devices, 5(4), 417–435. https://doi.org/10.1016/j.jsamd.2020.09.001
[3] Abate, A. R., Thiele, J., & Weitz, D. A. (2011). One-step formation of multiple emulsions in microfluidics. Lab on a Chip, 11(2), 253–258. https://doi.org/10.1039/c0lc00236d
[4] Chong, D. T., Liu, X. S., Ma, H. J., Huang, G. Y., Han, Y. L., Cui, X. Y., … Xu, F. (2015). Advances in fabricating double-emulsion droplets and their biomedical applications. Microfluidics and Nanofluidics, 19(5), 1071–1090. https://doi.org/10.1007/s10404-015-1635-8
[5] Ekanem, E. E. (2015). Author ’ s Accepted Manuscript Double emulsion production in glass capillary microfluidic device : Parametric investigation of droplet generation behaviour. https://doi.org/10.1016/j.ces.2015.03.004
[6] Kim, S. H., Kim, J. W., Cho, J. C., & Weitz, D. A. (2011). Double-emulsion drops with ultra-thin shells for capsule templates. Lab on a Chip, 11(18), 3162–3166. https://doi.org/10.1039/c1lc20434c